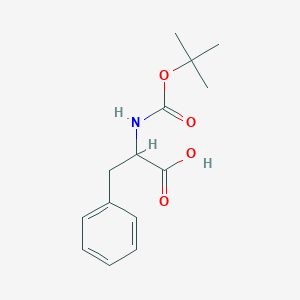
Boc-Phe-OH
Overview
Description
N-(tert-Butoxycarbonyl)-L-phenylalanine: It is a white crystalline solid that is stable at room temperature and soluble in organic solvents such as dimethylformamide and dichloromethane. The compound is primarily used as a protecting group for amino acids, which helps to prevent unwanted reactions during peptide synthesis.
Preparation Methods
Synthetic Routes and Reaction Conditions: Boc-Phe-OH is synthesized by reacting L-phenylalanine with di-tert-butyl dicarbonate in the presence of a base such as sodium hydroxide . The reaction typically occurs in an aqueous medium and results in the formation of this compound after purification .
Industrial Production Methods: In industrial settings, this compound is produced using solid-phase peptide synthesis (SPPS) techniques. This method involves the successive coupling of N-protected amino acids to a growing peptide chain immobilized on a solid support . The Boc strategy is favored for its stability and ease of removal under acidic conditions .
Chemical Reactions Analysis
Types of Reactions: Boc-Phe-OH undergoes various chemical reactions, including:
Substitution Reactions: The Boc group can be removed under acidic conditions, allowing for further functionalization of the amino acid.
Oxidation and Reduction: The phenylalanine moiety can undergo oxidation and reduction reactions, although these are less common in peptide synthesis.
Common Reagents and Conditions:
Acidic Conditions: Trifluoroacetic acid is commonly used to remove the Boc protecting group.
Oxidizing Agents: Hydrogen peroxide can be used for oxidation reactions.
Major Products Formed:
Deprotected Amino Acids: Removal of the Boc group yields free amino acids, which can be further utilized in peptide synthesis.
Scientific Research Applications
Boc-Phe-OH has a wide range of applications in scientific research:
Chemistry: It is used in the synthesis of peptides and proteins, serving as a protecting group for amino acids.
Biology: this compound is employed in the study of enzyme-substrate interactions and protein folding.
Medicine: The compound is used in the development of peptide-based drugs and therapeutic agents.
Industry: this compound is utilized in the production of biomaterials and nanostructures for drug delivery systems.
Mechanism of Action
The primary function of Boc-Phe-OH is to protect the amino group of phenylalanine during peptide synthesis. The Boc group is stable under basic conditions but can be easily removed under acidic conditions, allowing for selective deprotection and further functionalization . The phenylalanine moiety can interact with various molecular targets through hydrophobic interactions, contributing to the stability and activity of the synthesized peptides .
Comparison with Similar Compounds
- N-(tert-Butoxycarbonyl)-L-leucine (Boc-Leu-OH)
- N-(tert-Butoxycarbonyl)-L-valine (Boc-Val-OH)
- N-(tert-Butoxycarbonyl)-L-alanine (Boc-Ala-OH)
Comparison: Boc-Phe-OH is unique due to its phenylalanine moiety, which provides hydrophobic interactions that are crucial for the stability and activity of peptides . In contrast, Boc-Leu-OH, Boc-Val-OH, and Boc-Ala-OH have different side chains that offer varying degrees of hydrophobicity and steric hindrance . This makes this compound particularly useful in applications where strong hydrophobic interactions are required .
Properties
IUPAC Name |
(2S)-2-[(2-methylpropan-2-yl)oxycarbonylamino]-3-phenylpropanoic acid | |
---|---|---|
Source | PubChem | |
URL | https://pubchem.ncbi.nlm.nih.gov | |
Description | Data deposited in or computed by PubChem | |
InChI |
InChI=1S/C14H19NO4/c1-14(2,3)19-13(18)15-11(12(16)17)9-10-7-5-4-6-8-10/h4-8,11H,9H2,1-3H3,(H,15,18)(H,16,17)/t11-/m0/s1 | |
Source | PubChem | |
URL | https://pubchem.ncbi.nlm.nih.gov | |
Description | Data deposited in or computed by PubChem | |
InChI Key |
ZYJPUMXJBDHSIF-NSHDSACASA-N | |
Source | PubChem | |
URL | https://pubchem.ncbi.nlm.nih.gov | |
Description | Data deposited in or computed by PubChem | |
Canonical SMILES |
CC(C)(C)OC(=O)NC(CC1=CC=CC=C1)C(=O)O | |
Source | PubChem | |
URL | https://pubchem.ncbi.nlm.nih.gov | |
Description | Data deposited in or computed by PubChem | |
Isomeric SMILES |
CC(C)(C)OC(=O)N[C@@H](CC1=CC=CC=C1)C(=O)O | |
Source | PubChem | |
URL | https://pubchem.ncbi.nlm.nih.gov | |
Description | Data deposited in or computed by PubChem | |
Molecular Formula |
C14H19NO4 | |
Source | PubChem | |
URL | https://pubchem.ncbi.nlm.nih.gov | |
Description | Data deposited in or computed by PubChem | |
DSSTOX Substance ID |
DTXSID90884634 | |
Record name | L-Phenylalanine, N-[(1,1-dimethylethoxy)carbonyl]- | |
Source | EPA DSSTox | |
URL | https://comptox.epa.gov/dashboard/DTXSID90884634 | |
Description | DSSTox provides a high quality public chemistry resource for supporting improved predictive toxicology. | |
Molecular Weight |
265.30 g/mol | |
Source | PubChem | |
URL | https://pubchem.ncbi.nlm.nih.gov | |
Description | Data deposited in or computed by PubChem | |
CAS No. |
13734-34-4 | |
Record name | BOC-L-Phenylalanine | |
Source | CAS Common Chemistry | |
URL | https://commonchemistry.cas.org/detail?cas_rn=13734-34-4 | |
Description | CAS Common Chemistry is an open community resource for accessing chemical information. Nearly 500,000 chemical substances from CAS REGISTRY cover areas of community interest, including common and frequently regulated chemicals, and those relevant to high school and undergraduate chemistry classes. This chemical information, curated by our expert scientists, is provided in alignment with our mission as a division of the American Chemical Society. | |
Explanation | The data from CAS Common Chemistry is provided under a CC-BY-NC 4.0 license, unless otherwise stated. | |
Record name | L-Phenylalanine, N-((1,1-dimethylethoxy)carbonyl)- | |
Source | ChemIDplus | |
URL | https://pubchem.ncbi.nlm.nih.gov/substance/?source=chemidplus&sourceid=0013734344 | |
Description | ChemIDplus is a free, web search system that provides access to the structure and nomenclature authority files used for the identification of chemical substances cited in National Library of Medicine (NLM) databases, including the TOXNET system. | |
Record name | L-Phenylalanine, N-[(1,1-dimethylethoxy)carbonyl]- | |
Source | EPA Chemicals under the TSCA | |
URL | https://www.epa.gov/chemicals-under-tsca | |
Description | EPA Chemicals under the Toxic Substances Control Act (TSCA) collection contains information on chemicals and their regulations under TSCA, including non-confidential content from the TSCA Chemical Substance Inventory and Chemical Data Reporting. | |
Record name | L-Phenylalanine, N-[(1,1-dimethylethoxy)carbonyl]- | |
Source | EPA DSSTox | |
URL | https://comptox.epa.gov/dashboard/DTXSID90884634 | |
Description | DSSTox provides a high quality public chemistry resource for supporting improved predictive toxicology. | |
Record name | N-(tert-butoxycarbonyl)-L-phenylalanine | |
Source | European Chemicals Agency (ECHA) | |
URL | https://echa.europa.eu/substance-information/-/substanceinfo/100.033.899 | |
Description | The European Chemicals Agency (ECHA) is an agency of the European Union which is the driving force among regulatory authorities in implementing the EU's groundbreaking chemicals legislation for the benefit of human health and the environment as well as for innovation and competitiveness. | |
Explanation | Use of the information, documents and data from the ECHA website is subject to the terms and conditions of this Legal Notice, and subject to other binding limitations provided for under applicable law, the information, documents and data made available on the ECHA website may be reproduced, distributed and/or used, totally or in part, for non-commercial purposes provided that ECHA is acknowledged as the source: "Source: European Chemicals Agency, http://echa.europa.eu/". Such acknowledgement must be included in each copy of the material. ECHA permits and encourages organisations and individuals to create links to the ECHA website under the following cumulative conditions: Links can only be made to webpages that provide a link to the Legal Notice page. | |
Retrosynthesis Analysis
AI-Powered Synthesis Planning: Our tool employs the Template_relevance Pistachio, Template_relevance Bkms_metabolic, Template_relevance Pistachio_ringbreaker, Template_relevance Reaxys, Template_relevance Reaxys_biocatalysis model, leveraging a vast database of chemical reactions to predict feasible synthetic routes.
One-Step Synthesis Focus: Specifically designed for one-step synthesis, it provides concise and direct routes for your target compounds, streamlining the synthesis process.
Accurate Predictions: Utilizing the extensive PISTACHIO, BKMS_METABOLIC, PISTACHIO_RINGBREAKER, REAXYS, REAXYS_BIOCATALYSIS database, our tool offers high-accuracy predictions, reflecting the latest in chemical research and data.
Strategy Settings
Precursor scoring | Relevance Heuristic |
---|---|
Min. plausibility | 0.01 |
Model | Template_relevance |
Template Set | Pistachio/Bkms_metabolic/Pistachio_ringbreaker/Reaxys/Reaxys_biocatalysis |
Top-N result to add to graph | 6 |
Feasible Synthetic Routes
Q1: What is the molecular formula and weight of Boc-phenylalanine?
A1: The molecular formula of Boc-phenylalanine is C14H19NO4, and its molecular weight is 265.30 g/mol.
Q2: Is there any spectroscopic data available for Boc-phenylalanine?
A2: Yes, several research papers mention using spectroscopic techniques like IR, 1H NMR, 13C NMR, and even 19F NMR [] to characterize Boc-phenylalanine and its derivatives.
Q3: How stable is Boc-phenylalanine under acidic conditions?
A3: While Boc-phenylalanine is commonly used in peptide synthesis which requires deprotection with acids, a study found that a p-carbamoylmethylbenzyl ester linkage of Boc-phenylalanine to polystyrene resin demonstrated increased stability toward trifluoroacetic acid compared to traditional benzyl ester linkages [].
Q4: Can Boc-phenylalanine be used in the synthesis of chiral molecules?
A4: Absolutely! Researchers have employed Boc-phenylalanine as a starting material in the stereospecific synthesis of various chiral compounds, including (S)2-amino-3-cyclohexyl-(R)1-(2-furanyl)-propan-1-ol and (S)2-amino-3-cyclohexyl-(R)1-(2-thienyl)-propan-1-ol [].
Q5: What role does Boc-phenylalanine play in dynamic kinetic resolution?
A5: Boc-phenylalanine ethyl thioester has been successfully employed as a substrate in Alcalase-catalyzed dynamic kinetic resolutions with benzylamine, resulting in the efficient production of enantiomerically pure (S)-N-Boc-phenylalanine benzylamide [, ].
Q6: Have any computational studies been performed on Boc-phenylalanine?
A6: Yes, time-dependent density functional theory (TD-DFT) calculations were employed to simulate circular dichroism spectra and assign the absolute configuration of diastereomeric mixed (phthalocyaninato)(porphyrinato) rare earth double-decker complexes resolved using L-Boc-Phe-OH as a chiral resolving agent [].
Q7: How does modifying the structure of Boc-phenylalanine affect its biological activity?
A7: Replacing the amide bond in the Phe-APAA portion of the plasma kallikrein inhibitor PKSI-527, a Boc-phenylalanine derivative, with a CH2-NH bond resulted in a significant loss of inhibitory activity, highlighting the importance of the amide bond for its activity [].
Q8: What kind of formulations improve the stability and bioavailability of curcumin?
A8: Researchers found that incorporating curcumin into mPEG-PLA-BP (methoxy-poly(ethylene glycol)-block-poly(lactic acid) end-capped with Boc-phenylalanine) micelles significantly improved its stability in the bloodstream and increased its bioavailability compared to free curcumin or curcumin loaded in non-modified mPEG-PLA micelles [, ].
Q9: What analytical techniques are used to study Boc-phenylalanine in molecularly imprinted polymers?
A9: Several techniques are employed, including high-performance liquid chromatography (HPLC) to assess enantiomeric separation [, ], Raman spectroscopy to study template presence and binding kinetics [], and fluorescence microscopy for target binding characterization in imprinted nanofibers [].
Q10: How does incorporating curcumin into micelles affect its dissolution and solubility?
A10: Encapsulating curcumin within mPEG-PLA-BP micelles significantly enhances its solubility in aqueous solutions, addressing the inherent poor water solubility of curcumin and potentially leading to improved bioavailability [, ].
Disclaimer and Information on In-Vitro Research Products
Please be aware that all articles and product information presented on BenchChem are intended solely for informational purposes. The products available for purchase on BenchChem are specifically designed for in-vitro studies, which are conducted outside of living organisms. In-vitro studies, derived from the Latin term "in glass," involve experiments performed in controlled laboratory settings using cells or tissues. It is important to note that these products are not categorized as medicines or drugs, and they have not received approval from the FDA for the prevention, treatment, or cure of any medical condition, ailment, or disease. We must emphasize that any form of bodily introduction of these products into humans or animals is strictly prohibited by law. It is essential to adhere to these guidelines to ensure compliance with legal and ethical standards in research and experimentation.