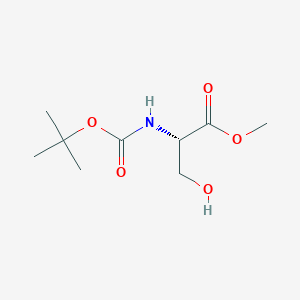
Boc-Ser-OMe
Overview
Description
N-(tert-Butoxycarbonyl)-L-serine methyl ester: , commonly known as Boc-Ser-OMe, is a derivative of the amino acid serine. It is widely used in peptide synthesis due to its stability and ease of handling. The compound has the molecular formula C9H17NO5 and a molecular weight of 219.23 g/mol .
Mechanism of Action
Target of Action
Boc-Ser-OMe, also known as N-(tert-Butoxycarbonyl)-L-serine methyl ester, is a derivative of the amino acid serine
Mode of Action
It’s known that this compound is used in peptide synthesis . The Boc group (tert-butoxycarbonyl) is a protective group used in organic synthesis. It prevents unwanted reactions at the amino group during peptide synthesis. After the desired reactions are complete, the Boc group can be removed under acidic conditions, revealing the original amino group .
Biochemical Pathways
Given its role in peptide synthesis, it may indirectly influence various biochemical pathways depending on the specific peptides being synthesized .
Result of Action
Its main role is to protect the amino group during synthesis and can be removed afterwards . Therefore, the results of its action are more related to the successful synthesis of the desired peptide.
Action Environment
The action of this compound is highly dependent on the conditions of the reaction it is used in. Factors such as pH, temperature, and the presence of other reactants can influence its efficacy and stability. For instance, the Boc group is stable under basic and neutral conditions but can be removed under acidic conditions .
Biochemical Analysis
Biochemical Properties
Boc-Ser-OMe plays a significant role in biochemical reactions, particularly in the formation of peptides. It is used in Boc solid-phase peptide synthesis, a method for creating specific peptide sequences
Cellular Effects
It is known that this compound is used in peptide synthesis, which can influence cell function, including impacts on cell signaling pathways, gene expression, and cellular metabolism .
Molecular Mechanism
The molecular mechanism of this compound primarily involves its role in peptide synthesis. It is used as a protective group in the synthesis of peptides, preventing unwanted side reactions . The compound exerts its effects at the molecular level through its interactions with other biomolecules during peptide synthesis .
Temporal Effects in Laboratory Settings
It is known that this compound is stable towards most nucleophiles and bases .
Metabolic Pathways
It is known that this compound is used in peptide synthesis, which is a crucial metabolic process .
Preparation Methods
Synthetic Routes and Reaction Conditions: Boc-Ser-OMe can be synthesized from L-serine through a series of chemical reactions. The process typically involves the protection of the amino group of serine with a tert-butoxycarbonyl (Boc) group and the esterification of the carboxyl group with methanol. The reaction conditions often include the use of bases and anhydrides such as Boc2O .
Industrial Production Methods: Industrial production of this compound follows similar synthetic routes but on a larger scale. The process is optimized for higher yields and purity, often involving automated systems for precise control of reaction conditions .
Chemical Reactions Analysis
Types of Reactions: Boc-Ser-OMe undergoes various chemical reactions, including:
Oxidation: Conversion to corresponding oxo compounds.
Reduction: Reduction of ester groups to alcohols.
Substitution: Nucleophilic substitution reactions where the Boc group can be replaced.
Common Reagents and Conditions:
Oxidation: Reagents like potassium permanganate (KMnO4) or chromium trioxide (CrO3).
Reduction: Reagents such as lithium aluminum hydride (LiAlH4).
Substitution: Conditions often involve strong nucleophiles and bases.
Major Products: The major products formed from these reactions depend on the specific conditions and reagents used. For example, oxidation can yield oxo compounds, while reduction can produce alcohols .
Scientific Research Applications
Boc-Ser-OMe is extensively used in scientific research, particularly in:
Chemistry: As a building block in peptide synthesis.
Biology: In the study of protein structure and function.
Medicine: For the development of peptide-based drugs.
Comparison with Similar Compounds
- N-(tert-Butoxycarbonyl)-L-cysteine methyl ester
- N-(tert-Butoxycarbonyl)-D-serine methyl ester
- N-Z-L-serine methyl ester
Uniqueness: Boc-Ser-OMe is unique due to its specific protective group (Boc) and esterification, which provide stability and ease of handling in peptide synthesis. Its properties make it particularly suitable for solid-phase peptide synthesis .
Biological Activity
Boc-Ser-OMe, or N-tert-butoxycarbonyl L-serine methyl ester, is a derivative of serine that has garnered attention in biochemical research due to its enzymatic and synthetic applications. This article explores the biological activity of this compound, focusing on its enzymatic interactions, synthesis, and potential applications in peptide synthesis and modification.
Overview of this compound
This compound is characterized by the presence of a tert-butoxycarbonyl (Boc) protecting group and a methyl ester functional group. This configuration enhances its stability and solubility, making it a suitable substrate for various enzymatic reactions.
Transgalactosylation Reactions
One of the significant biological activities of this compound is its role as an acceptor in transgalactosylation reactions catalyzed by β-galactosidase from Escherichia coli. The enzyme facilitates the transfer of galactose from donor substrates such as lactose to serine derivatives. Research has shown that this compound exhibits higher initial reaction rates and bioconversion yields compared to other serine derivatives like N-Z-Ser-OMe.
Table 1: Bioconversion Yields of Serine Derivatives in Transgalactosylation
Serine Derivative | Initial Rate (μmol/L.h) | Bioconversion Yield (%) |
---|---|---|
N-Boc-Ser-OMe | 7816.8 | 15.00 |
N-Z-Ser-OMe | 4680.0 | 8.00 |
Fmoc-Ser-OH | 1731.2 | 5.62 |
The optimal conditions for these reactions were identified as a heptane:buffer mixture (70:30) with a molar ratio of sugar to serine derivative at 1:3. The preference for this compound over other derivatives highlights its superior affinity for the enzyme's active site, leading to enhanced product formation rates .
Synthesis and Characterization
This compound can be synthesized through several methods, including solid-phase peptide synthesis (SPPS). The Boc protecting group can be removed under acidic conditions to yield free serine, which can then be utilized in further peptide synthesis or modification processes.
Case Study: Peptide Synthesis
In a study focusing on glycopeptides, this compound was used as a building block in constructing more complex peptide structures. The incorporation of this compound allowed for the controlled introduction of serine residues into peptides, facilitating studies on glycosylation effects on peptide conformation .
Table 2: Effects of Glycosylation on Peptide Conformation
Peptide Structure | Conformational Change Observed |
---|---|
Boc-Asn(GlcNAc)-Gly-Ser-OMe | Significant |
Boc-Asn-Gly-Ser-NHMe | Minimal |
The study indicated that glycosylation could significantly alter the structural properties of peptides containing this compound, emphasizing its importance in biochemical research.
Applications and Future Directions
The biological activity of this compound extends beyond simple enzymatic reactions. Its ability to participate in transgalactosylation makes it a valuable tool in synthesizing glycosylated peptides, which have applications in drug development and therapeutic protein engineering.
Future research may explore:
- Expanded Enzymatic Applications : Investigating other enzymes that can utilize this compound as an acceptor for various glycosyl donors.
- Peptide Therapeutics : Developing novel peptides incorporating this compound to enhance bioactivity and stability.
- Analytical Techniques : Utilizing mass spectrometry and NMR spectroscopy to further elucidate the structural characteristics and dynamics of peptides containing this compound.
Properties
IUPAC Name |
methyl (2S)-3-hydroxy-2-[(2-methylpropan-2-yl)oxycarbonylamino]propanoate | |
---|---|---|
Source | PubChem | |
URL | https://pubchem.ncbi.nlm.nih.gov | |
Description | Data deposited in or computed by PubChem | |
InChI |
InChI=1S/C9H17NO5/c1-9(2,3)15-8(13)10-6(5-11)7(12)14-4/h6,11H,5H2,1-4H3,(H,10,13)/t6-/m0/s1 | |
Source | PubChem | |
URL | https://pubchem.ncbi.nlm.nih.gov | |
Description | Data deposited in or computed by PubChem | |
InChI Key |
SANNKFASHWONFD-LURJTMIESA-N | |
Source | PubChem | |
URL | https://pubchem.ncbi.nlm.nih.gov | |
Description | Data deposited in or computed by PubChem | |
Canonical SMILES |
CC(C)(C)OC(=O)NC(CO)C(=O)OC | |
Source | PubChem | |
URL | https://pubchem.ncbi.nlm.nih.gov | |
Description | Data deposited in or computed by PubChem | |
Isomeric SMILES |
CC(C)(C)OC(=O)N[C@@H](CO)C(=O)OC | |
Source | PubChem | |
URL | https://pubchem.ncbi.nlm.nih.gov | |
Description | Data deposited in or computed by PubChem | |
Molecular Formula |
C9H17NO5 | |
Source | PubChem | |
URL | https://pubchem.ncbi.nlm.nih.gov | |
Description | Data deposited in or computed by PubChem | |
DSSTOX Substance ID |
DTXSID30427089 | |
Record name | Boc-Ser-OMe | |
Source | EPA DSSTox | |
URL | https://comptox.epa.gov/dashboard/DTXSID30427089 | |
Description | DSSTox provides a high quality public chemistry resource for supporting improved predictive toxicology. | |
Molecular Weight |
219.23 g/mol | |
Source | PubChem | |
URL | https://pubchem.ncbi.nlm.nih.gov | |
Description | Data deposited in or computed by PubChem | |
CAS No. |
2766-43-0 | |
Record name | Boc-Ser-OMe | |
Source | EPA DSSTox | |
URL | https://comptox.epa.gov/dashboard/DTXSID30427089 | |
Description | DSSTox provides a high quality public chemistry resource for supporting improved predictive toxicology. | |
Record name | N-(tert-BUTOXYCARBONYL)-L-SERINE METHYL ESTER | |
Source | European Chemicals Agency (ECHA) | |
URL | https://echa.europa.eu/information-on-chemicals | |
Description | The European Chemicals Agency (ECHA) is an agency of the European Union which is the driving force among regulatory authorities in implementing the EU's groundbreaking chemicals legislation for the benefit of human health and the environment as well as for innovation and competitiveness. | |
Explanation | Use of the information, documents and data from the ECHA website is subject to the terms and conditions of this Legal Notice, and subject to other binding limitations provided for under applicable law, the information, documents and data made available on the ECHA website may be reproduced, distributed and/or used, totally or in part, for non-commercial purposes provided that ECHA is acknowledged as the source: "Source: European Chemicals Agency, http://echa.europa.eu/". Such acknowledgement must be included in each copy of the material. ECHA permits and encourages organisations and individuals to create links to the ECHA website under the following cumulative conditions: Links can only be made to webpages that provide a link to the Legal Notice page. | |
Retrosynthesis Analysis
AI-Powered Synthesis Planning: Our tool employs the Template_relevance Pistachio, Template_relevance Bkms_metabolic, Template_relevance Pistachio_ringbreaker, Template_relevance Reaxys, Template_relevance Reaxys_biocatalysis model, leveraging a vast database of chemical reactions to predict feasible synthetic routes.
One-Step Synthesis Focus: Specifically designed for one-step synthesis, it provides concise and direct routes for your target compounds, streamlining the synthesis process.
Accurate Predictions: Utilizing the extensive PISTACHIO, BKMS_METABOLIC, PISTACHIO_RINGBREAKER, REAXYS, REAXYS_BIOCATALYSIS database, our tool offers high-accuracy predictions, reflecting the latest in chemical research and data.
Strategy Settings
Precursor scoring | Relevance Heuristic |
---|---|
Min. plausibility | 0.01 |
Model | Template_relevance |
Template Set | Pistachio/Bkms_metabolic/Pistachio_ringbreaker/Reaxys/Reaxys_biocatalysis |
Top-N result to add to graph | 6 |
Feasible Synthetic Routes
Q1: Why is Boc-Ser-OMe often used as a starting material in peptide synthesis?
A1: this compound is frequently utilized in peptide synthesis as a building block due to the presence of protecting groups on both the amine and carboxyl functionalities of serine. The Boc (tert-butyloxycarbonyl) group protects the amine, while the methyl ester protects the carboxyl group. These protecting groups are crucial for controlling the reactivity of the amino acid during peptide chain elongation. [, ]
Q2: Can you elaborate on the use of this compound in glycosylation reactions?
A2: this compound serves as an effective acceptor molecule in enzymatic glycosylation reactions using β-galactosidase. [] This process involves the transfer of a galactose sugar molecule from a donor, such as lactose, to the hydroxyl group of serine. The reaction conditions, including lactose concentration and temperature, significantly impact the yield of mono- and di-galactosylated products. []
Q3: Are there any studies on alternative phosphitylating agents for this compound in phosphopeptide synthesis?
A3: Yes, research has explored N, N-diisopropyl-bis(4-chlorobenzyl) phosphoramidite as an alternative phosphitylating agent for this compound. [] This reagent enables the efficient phosphorylation of the hydroxyl group in this compound, leading to the formation of protected phosphopeptides. Notably, this approach allows for the selective removal of the Boc group or the 4-chlorobenzyl protecting groups on the phosphate, providing flexibility in subsequent synthetic steps. []
Disclaimer and Information on In-Vitro Research Products
Please be aware that all articles and product information presented on BenchChem are intended solely for informational purposes. The products available for purchase on BenchChem are specifically designed for in-vitro studies, which are conducted outside of living organisms. In-vitro studies, derived from the Latin term "in glass," involve experiments performed in controlled laboratory settings using cells or tissues. It is important to note that these products are not categorized as medicines or drugs, and they have not received approval from the FDA for the prevention, treatment, or cure of any medical condition, ailment, or disease. We must emphasize that any form of bodily introduction of these products into humans or animals is strictly prohibited by law. It is essential to adhere to these guidelines to ensure compliance with legal and ethical standards in research and experimentation.