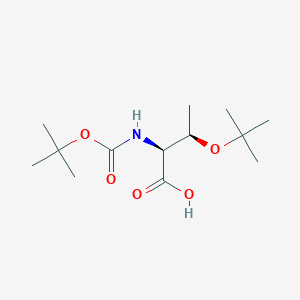
Boc-Thr(tBu)-OH
Overview
Description
“Boc-Thr(tBu)-OH” is a threonine derivative . It is used in peptide synthesis . The full name of this compound is "(S)-3-tert-Butoxy-2-tert-butoxycarbonylamino-butyric acid, Boc-O-tert-butyl-L-threonine" .
Synthesis Analysis
“this compound” participates in the synthesis of 2,3-unsaturated glycosides, via reaction with per-O-acetylated glucal in the presence of Er(OTf)3 catalyst .Molecular Structure Analysis
The linear formula of “this compound” is (CH3)3COCH(CH3)CH(COOH)NHCOOC(CH3)3 . It has a molecular weight of 275.34 .Chemical Reactions Analysis
“this compound” is used in peptide synthesis . It is also used as a building block for the introduction of N-terminal threonine amino-acid residues by Fmoc SPPS .Physical And Chemical Properties Analysis
“this compound” is a white to slight yellow to beige powder . It has a melting point of 95-98 °C . It is soluble in DMF .Scientific Research Applications
Synthesis of Protected Glycopeptides : Boc-Thr(tBu)-OH is used in the synthesis of protected glycopeptides, as demonstrated in the synthesis of a protected glycotetrapeptide corresponding to the N-terminal amino acid sequence of a specific peptide. This application is vital in peptide chemistry and drug design (Gobbo et al., 1988).
Peptide Synthesis Side Reactions : In another study, this compound was used in the synthesis of a double-chain peptide, where it was observed that certain synthesis conditions could lead to side reactions such as trifluoroacetylation (Hübener et al., 1992).
Manufacturing of Hydrophobic Peptides : this compound was also used in the synthesis of hydrophobic peptides, which are common in cancer vaccinations. Its application in large-scale production addresses challenges in peptide industry (Shakoori & Gangakhedkar, 2014).
Development of Phosphothreonine Derivatives : In peptide synthesis, this compound is utilized for creating phosphothreonine derivatives, which are important for studying calcium phosphate binding in peptides (Perich et al., 2009).
Synthesis of Kinin Analogues : The compound is also used in the synthesis of linear and cyclic kinin analogues, which have potential biological activity and pharmaceutical applications (Gobbo et al., 2009).
Bifunctional Peptides : It plays a role in the synthesis of bifunctional peptides that have opioid agonist and substance P antagonist bioactivities, a novel approach in pain management (Yamamoto et al., 2008).
Mechanism of Action
Target of Action
The primary target of Boc-Thr(tBu)-OH is the peptide chain in peptide synthesis . It acts as a protecting group for the amino acid threonine during the synthesis process .
Mode of Action
this compound interacts with its target by attaching to the amino acid threonine, protecting it from reacting with other compounds during the synthesis process . This protection allows for the controlled assembly of the peptide chain .
Biochemical Pathways
The use of this compound affects the pathway of peptide synthesis. It allows for the stepwise construction of the peptide chain, with each amino acid being added in a controlled manner . The protecting group is then removed in a deprotection step, allowing the peptide chain to be completed .
Pharmacokinetics
As a chemical used in peptide synthesis, this compound does not have traditional pharmacokinetic properties such as absorption, distribution, metabolism, and excretion (ADME). Instead, its impact on bioavailability is related to its role in peptide synthesis. By protecting the amino acid threonine, it ensures that the peptide chain is assembled correctly, which is crucial for the bioactivity of the final peptide product .
Result of Action
The molecular effect of this compound’s action is the successful synthesis of a peptide with the correct sequence of amino acids . On a cellular level, the peptides synthesized using this compound can have various effects depending on their specific sequences and the cells they interact with .
Action Environment
The action of this compound is influenced by the conditions of the peptide synthesis process. Factors such as temperature, pH, and the presence of other chemicals can affect its efficacy and stability . For example, the removal of the protecting group requires the use of specific reagents and reaction conditions .
Safety and Hazards
properties
IUPAC Name |
(2S,3R)-3-[(2-methylpropan-2-yl)oxy]-2-[(2-methylpropan-2-yl)oxycarbonylamino]butanoic acid | |
---|---|---|
Source | PubChem | |
URL | https://pubchem.ncbi.nlm.nih.gov | |
Description | Data deposited in or computed by PubChem | |
InChI |
InChI=1S/C13H25NO5/c1-8(18-12(2,3)4)9(10(15)16)14-11(17)19-13(5,6)7/h8-9H,1-7H3,(H,14,17)(H,15,16)/t8-,9+/m1/s1 | |
Source | PubChem | |
URL | https://pubchem.ncbi.nlm.nih.gov | |
Description | Data deposited in or computed by PubChem | |
InChI Key |
LKRXXARJBFBMCE-BDAKNGLRSA-N | |
Source | PubChem | |
URL | https://pubchem.ncbi.nlm.nih.gov | |
Description | Data deposited in or computed by PubChem | |
Canonical SMILES |
CC(C(C(=O)O)NC(=O)OC(C)(C)C)OC(C)(C)C | |
Source | PubChem | |
URL | https://pubchem.ncbi.nlm.nih.gov | |
Description | Data deposited in or computed by PubChem | |
Isomeric SMILES |
C[C@H]([C@@H](C(=O)O)NC(=O)OC(C)(C)C)OC(C)(C)C | |
Source | PubChem | |
URL | https://pubchem.ncbi.nlm.nih.gov | |
Description | Data deposited in or computed by PubChem | |
Molecular Formula |
C13H25NO5 | |
Source | PubChem | |
URL | https://pubchem.ncbi.nlm.nih.gov | |
Description | Data deposited in or computed by PubChem | |
DSSTOX Substance ID |
DTXSID40426760 | |
Record name | Boc-Thr(tBu)-OH | |
Source | EPA DSSTox | |
URL | https://comptox.epa.gov/dashboard/DTXSID40426760 | |
Description | DSSTox provides a high quality public chemistry resource for supporting improved predictive toxicology. | |
Molecular Weight |
275.34 g/mol | |
Source | PubChem | |
URL | https://pubchem.ncbi.nlm.nih.gov | |
Description | Data deposited in or computed by PubChem | |
CAS RN |
13734-40-2 | |
Record name | N-[(1,1-Dimethylethoxy)carbonyl]-O-(1,1-dimethylethyl)-L-threonine | |
Source | CAS Common Chemistry | |
URL | https://commonchemistry.cas.org/detail?cas_rn=13734-40-2 | |
Description | CAS Common Chemistry is an open community resource for accessing chemical information. Nearly 500,000 chemical substances from CAS REGISTRY cover areas of community interest, including common and frequently regulated chemicals, and those relevant to high school and undergraduate chemistry classes. This chemical information, curated by our expert scientists, is provided in alignment with our mission as a division of the American Chemical Society. | |
Explanation | The data from CAS Common Chemistry is provided under a CC-BY-NC 4.0 license, unless otherwise stated. | |
Record name | Boc-Thr(tBu)-OH | |
Source | EPA DSSTox | |
URL | https://comptox.epa.gov/dashboard/DTXSID40426760 | |
Description | DSSTox provides a high quality public chemistry resource for supporting improved predictive toxicology. | |
Record name | (2S,3R)-3-(tert-butoxy)-2-{[(tert-butoxy)carbonyl]amino}butanoic acid | |
Source | European Chemicals Agency (ECHA) | |
URL | https://echa.europa.eu/information-on-chemicals | |
Description | The European Chemicals Agency (ECHA) is an agency of the European Union which is the driving force among regulatory authorities in implementing the EU's groundbreaking chemicals legislation for the benefit of human health and the environment as well as for innovation and competitiveness. | |
Explanation | Use of the information, documents and data from the ECHA website is subject to the terms and conditions of this Legal Notice, and subject to other binding limitations provided for under applicable law, the information, documents and data made available on the ECHA website may be reproduced, distributed and/or used, totally or in part, for non-commercial purposes provided that ECHA is acknowledged as the source: "Source: European Chemicals Agency, http://echa.europa.eu/". Such acknowledgement must be included in each copy of the material. ECHA permits and encourages organisations and individuals to create links to the ECHA website under the following cumulative conditions: Links can only be made to webpages that provide a link to the Legal Notice page. | |
Retrosynthesis Analysis
AI-Powered Synthesis Planning: Our tool employs the Template_relevance Pistachio, Template_relevance Bkms_metabolic, Template_relevance Pistachio_ringbreaker, Template_relevance Reaxys, Template_relevance Reaxys_biocatalysis model, leveraging a vast database of chemical reactions to predict feasible synthetic routes.
One-Step Synthesis Focus: Specifically designed for one-step synthesis, it provides concise and direct routes for your target compounds, streamlining the synthesis process.
Accurate Predictions: Utilizing the extensive PISTACHIO, BKMS_METABOLIC, PISTACHIO_RINGBREAKER, REAXYS, REAXYS_BIOCATALYSIS database, our tool offers high-accuracy predictions, reflecting the latest in chemical research and data.
Strategy Settings
Precursor scoring | Relevance Heuristic |
---|---|
Min. plausibility | 0.01 |
Model | Template_relevance |
Template Set | Pistachio/Bkms_metabolic/Pistachio_ringbreaker/Reaxys/Reaxys_biocatalysis |
Top-N result to add to graph | 6 |
Feasible Synthetic Routes
Disclaimer and Information on In-Vitro Research Products
Please be aware that all articles and product information presented on BenchChem are intended solely for informational purposes. The products available for purchase on BenchChem are specifically designed for in-vitro studies, which are conducted outside of living organisms. In-vitro studies, derived from the Latin term "in glass," involve experiments performed in controlled laboratory settings using cells or tissues. It is important to note that these products are not categorized as medicines or drugs, and they have not received approval from the FDA for the prevention, treatment, or cure of any medical condition, ailment, or disease. We must emphasize that any form of bodily introduction of these products into humans or animals is strictly prohibited by law. It is essential to adhere to these guidelines to ensure compliance with legal and ethical standards in research and experimentation.