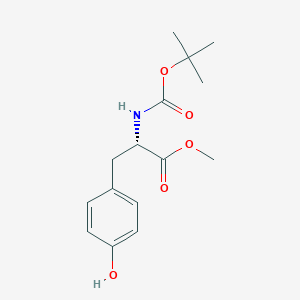
Boc-Tyr-OMe
Overview
Description
Boc-Tyr-OMe, also known as N-(tert-Butoxycarbonyl)-L-tyrosine Methyl Ester or Boc-L-tyrosine methyl ester, is a derivative of tyrosine . It has a molecular weight of 295.34 and a chemical formula of C₁₅H₂₁NO₅ .
Synthesis Analysis
Boc-Tyr-OMe can be synthesized through various methods. One such method involves the synthesis of dipeptides with tyrosine and methionine in the presence of triazine . Tripeptides are then prepared using the same method with valine and alanine amino acids. New methacrylate monomers are synthesized by the reaction of phenolic OH of tyrosine in tripeptides and methacryloyl chloride .
Molecular Structure Analysis
The molecular structure of Boc-Tyr-OMe is represented by the formula C₁₅H₂₁NO₅ . It is a complex molecule with a specific arrangement of atoms and bonds.
Chemical Reactions Analysis
Boc-Tyr-OMe is suitable for Boc solid-phase peptide synthesis . This type of synthesis is a common method used in the production of peptides.
Physical And Chemical Properties Analysis
Boc-Tyr-OMe is a solid substance with a molecular weight of 295.33 . It has a melting point of 100-104 °C . The optical activity of Boc-Tyr-OMe is [α]22/D +51°, c = 1 in chloroform .
Scientific Research Applications
Boc-Tyr-OMe in Scientific Research Applications
Peptide Synthesis: Boc-Tyr-OMe is commonly used in peptide synthesis as a protected amino acid. The Boc (tert-butoxycarbonyl) group protects the amino group during the synthesis process, allowing for the selective formation of peptide bonds without unwanted side reactions. This compound is particularly useful in solid-phase peptide synthesis (SPPS), where it can be coupled onto a resin and then used to sequentially build a peptide chain .
Enzymatic Peptide Synthesis: In enzymatic peptide synthesis, Boc-Tyr-OMe serves as a substrate for enzymes like carboxypeptidase, which can catalyze the formation of dipeptides. This method is used to produce specific dipeptide precursors, such as BOC-Tyr-Ala, which are important in various biochemical processes and have potential therapeutic applications .
Oxidative Functionalization: Boc-Tyr-OMe is also involved in oxidative functionalization reactions mediated by enzymes like tyrosinase. These reactions are crucial for the synthesis of DOPA-derived peptidomimetics, which are compounds that mimic the structure of peptides and have applications in treating diseases like Parkinson’s disease .
Chiral Building Blocks: As a chiral building block, Boc-Tyr-OMe is used to introduce chirality into synthetic molecules. Chirality is a key factor in the biological activity of many drugs, and using chiral building blocks like Boc-Tyr-OMe can help create more effective pharmaceutical compounds .
Phenol Derivative Synthesis: Boc-Tyr-OMe is used in the selective generation of ortho-brominated phenol derivatives under certain conditions. These derivatives have various applications, including as intermediates in organic synthesis and potential use in pharmaceuticals .
Research and Development: In the broader context of research and development, Boc-Tyr-OMe is a valuable reagent for exploring new synthetic pathways and developing novel compounds with potential applications across different fields of science and technology .
Safety and Hazards
Future Directions
Boc-Tyr-OMe is primarily used for research purposes . It is a key component in the synthesis of peptides, which have a wide range of applications in biological and medicinal research. The future directions of Boc-Tyr-OMe will likely continue to be in the field of peptide synthesis and related research areas.
Mechanism of Action
Target of Action
Boc-Tyr-OMe, also known as N-(tert-Butoxycarbonyl)-L-tyrosine methyl ester or Boc-L-tyrosine methyl ester , is a derivative of the amino acid tyrosine. It is primarily used in peptide synthesis . The primary targets of Boc-Tyr-OMe are the enzymes and proteins involved in peptide synthesis, where it serves as a building block.
Mode of Action
Boc-Tyr-OMe interacts with its targets through the process of peptide synthesis. It is incorporated into the growing peptide chain during the synthesis process. The Boc group in Boc-Tyr-OMe serves as a protective group for the amino group during peptide synthesis, preventing unwanted side reactions .
Biochemical Pathways
Boc-Tyr-OMe is involved in the biochemical pathway of peptide synthesis. It is used as a building block in the formation of peptides, which are chains of amino acids linked by peptide bonds. The Boc group in Boc-Tyr-OMe is removed during the final steps of peptide synthesis, revealing the amino group that can then participate in the formation of peptide bonds .
Result of Action
The result of Boc-Tyr-OMe’s action is the successful synthesis of peptides. By serving as a building block in peptide synthesis and protecting the amino group from unwanted side reactions, Boc-Tyr-OMe contributes to the formation of peptides with the desired sequence and structure .
Action Environment
The action of Boc-Tyr-OMe is influenced by various environmental factors. For instance, the efficiency of peptide synthesis can be affected by factors such as temperature, pH, and the presence of other substances in the reaction mixture . Furthermore, the stability of Boc-Tyr-OMe can be influenced by factors such as temperature, light, and the presence of moisture .
properties
IUPAC Name |
methyl (2S)-3-(4-hydroxyphenyl)-2-[(2-methylpropan-2-yl)oxycarbonylamino]propanoate | |
---|---|---|
Source | PubChem | |
URL | https://pubchem.ncbi.nlm.nih.gov | |
Description | Data deposited in or computed by PubChem | |
InChI |
InChI=1S/C15H21NO5/c1-15(2,3)21-14(19)16-12(13(18)20-4)9-10-5-7-11(17)8-6-10/h5-8,12,17H,9H2,1-4H3,(H,16,19)/t12-/m0/s1 | |
Source | PubChem | |
URL | https://pubchem.ncbi.nlm.nih.gov | |
Description | Data deposited in or computed by PubChem | |
InChI Key |
NQIFXJSLCUJHBB-LBPRGKRZSA-N | |
Source | PubChem | |
URL | https://pubchem.ncbi.nlm.nih.gov | |
Description | Data deposited in or computed by PubChem | |
Canonical SMILES |
CC(C)(C)OC(=O)NC(CC1=CC=C(C=C1)O)C(=O)OC | |
Source | PubChem | |
URL | https://pubchem.ncbi.nlm.nih.gov | |
Description | Data deposited in or computed by PubChem | |
Isomeric SMILES |
CC(C)(C)OC(=O)N[C@@H](CC1=CC=C(C=C1)O)C(=O)OC | |
Source | PubChem | |
URL | https://pubchem.ncbi.nlm.nih.gov | |
Description | Data deposited in or computed by PubChem | |
Molecular Formula |
C15H21NO5 | |
Source | PubChem | |
URL | https://pubchem.ncbi.nlm.nih.gov | |
Description | Data deposited in or computed by PubChem | |
DSSTOX Substance ID |
DTXSID60427077 | |
Record name | Boc-Tyr-OMe | |
Source | EPA DSSTox | |
URL | https://comptox.epa.gov/dashboard/DTXSID60427077 | |
Description | DSSTox provides a high quality public chemistry resource for supporting improved predictive toxicology. | |
Molecular Weight |
295.33 g/mol | |
Source | PubChem | |
URL | https://pubchem.ncbi.nlm.nih.gov | |
Description | Data deposited in or computed by PubChem | |
Product Name |
Boc-Tyr-OMe | |
CAS RN |
4326-36-7 | |
Record name | Boc-Tyr-OMe | |
Source | EPA DSSTox | |
URL | https://comptox.epa.gov/dashboard/DTXSID60427077 | |
Description | DSSTox provides a high quality public chemistry resource for supporting improved predictive toxicology. | |
Record name | Boc-Tyr-OMe | |
Source | European Chemicals Agency (ECHA) | |
URL | https://echa.europa.eu/information-on-chemicals | |
Description | The European Chemicals Agency (ECHA) is an agency of the European Union which is the driving force among regulatory authorities in implementing the EU's groundbreaking chemicals legislation for the benefit of human health and the environment as well as for innovation and competitiveness. | |
Explanation | Use of the information, documents and data from the ECHA website is subject to the terms and conditions of this Legal Notice, and subject to other binding limitations provided for under applicable law, the information, documents and data made available on the ECHA website may be reproduced, distributed and/or used, totally or in part, for non-commercial purposes provided that ECHA is acknowledged as the source: "Source: European Chemicals Agency, http://echa.europa.eu/". Such acknowledgement must be included in each copy of the material. ECHA permits and encourages organisations and individuals to create links to the ECHA website under the following cumulative conditions: Links can only be made to webpages that provide a link to the Legal Notice page. | |
Synthesis routes and methods
Procedure details
Retrosynthesis Analysis
AI-Powered Synthesis Planning: Our tool employs the Template_relevance Pistachio, Template_relevance Bkms_metabolic, Template_relevance Pistachio_ringbreaker, Template_relevance Reaxys, Template_relevance Reaxys_biocatalysis model, leveraging a vast database of chemical reactions to predict feasible synthetic routes.
One-Step Synthesis Focus: Specifically designed for one-step synthesis, it provides concise and direct routes for your target compounds, streamlining the synthesis process.
Accurate Predictions: Utilizing the extensive PISTACHIO, BKMS_METABOLIC, PISTACHIO_RINGBREAKER, REAXYS, REAXYS_BIOCATALYSIS database, our tool offers high-accuracy predictions, reflecting the latest in chemical research and data.
Strategy Settings
Precursor scoring | Relevance Heuristic |
---|---|
Min. plausibility | 0.01 |
Model | Template_relevance |
Template Set | Pistachio/Bkms_metabolic/Pistachio_ringbreaker/Reaxys/Reaxys_biocatalysis |
Top-N result to add to graph | 6 |
Feasible Synthetic Routes
Disclaimer and Information on In-Vitro Research Products
Please be aware that all articles and product information presented on BenchChem are intended solely for informational purposes. The products available for purchase on BenchChem are specifically designed for in-vitro studies, which are conducted outside of living organisms. In-vitro studies, derived from the Latin term "in glass," involve experiments performed in controlled laboratory settings using cells or tissues. It is important to note that these products are not categorized as medicines or drugs, and they have not received approval from the FDA for the prevention, treatment, or cure of any medical condition, ailment, or disease. We must emphasize that any form of bodily introduction of these products into humans or animals is strictly prohibited by law. It is essential to adhere to these guidelines to ensure compliance with legal and ethical standards in research and experimentation.