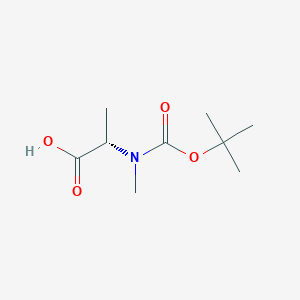
Boc-N-Me-Ala-OH
Overview
Description
Boc-N-Me-Ala-OH (N-(tert-butoxycarbonyl)-N-methyl-L-alanine) is a protected amino acid derivative widely used in peptide synthesis. Its structure features a tert-butoxycarbonyl (Boc) protecting group on the α-amino group and a methyl group on the amide nitrogen, rendering it resistant to nucleophilic attack and enzymatic degradation during solid-phase peptide synthesis (SPPS) .
Preparation Methods
Synthetic Route via Direct Boc Protection of N-Methyl-L-Alanine
The most straightforward method for synthesizing Boc-N-Me-Ala-OH involves the direct protection of commercially available N-methyl-L-alanine (H-MeAla-OH) using di-tert-butyl dicarbonate [(Boc)₂O]. This approach mirrors the protection of standard amino acids but requires adjustments to account for the steric and electronic effects of the N-methyl group.
Reaction Conditions and Procedure
-
Base Selection : Aqueous sodium hydroxide (NaOH) is employed to deprotonate the amino group, facilitating nucleophilic attack on (Boc)₂O.
-
Solvent System : A biphasic mixture of tetrahydrofuran (THF) and water enhances solubility while maintaining reaction efficiency .
-
Stoichiometry :
Procedure :
-
Dissolve H-MeAla-OH (10.0 g, 89.5 mmol) in H₂O (56 mL) and THF (56 mL).
-
Add NaOH (6.73 g, 168 mmol) at 0°C, followed by dropwise addition of (Boc)₂O (31.85 g, 146 mmol).
-
Stir at room temperature for 17 hours.
-
Extract unreacted reagents with petroleum ether (2 × 100 mL).
-
Acidify the aqueous layer to pH 1–2 using 4 M HCl, then extract the product with ethyl acetate (4 × 100 mL).
-
Dry the organic phase over Na₂SO₄, filter, and concentrate under reduced pressure to yield this compound as a colorless oil .
Yield : ~95–98% (crude), depending on purification .
Optimization of Reaction Parameters
Solvent Effects
The choice of solvent significantly impacts reaction efficiency. Polar aprotic solvents like THF improve Boc group transfer by stabilizing the transition state, while water ensures solubility of the amino acid. Mixed solvent systems (THF:H₂O = 1:1) are optimal for balancing reactivity and solubility .
Temperature and Time
Room temperature (20–25°C) is sufficient for complete Boc protection within 17 hours. Elevated temperatures (40–50°C) reduce reaction time to 6–8 hours but risk racemization or side reactions .
Base Compatibility
While NaOH is standard, alternative bases like NaHCO₃ or triethylamine (TEA) may be used in organic phases. However, aqueous NaOH remains preferred for its ability to maintain high pH without phase separation .
Characterization and Quality Control
Spectroscopic Analysis
-
¹H NMR (CDCl₃) :
-
FTIR :
-
1730 cm⁻¹ (C=O, Boc carbonyl)
-
1680 cm⁻¹ (C=O, carboxylic acid).
-
Purity Assessment
-
HPLC : Reverse-phase C18 column (gradient: 5–95% acetonitrile in H₂O + 0.1% TFA) confirms purity >98% .
-
Melting Point : 79–83°C (lit.), though the compound is often isolated as an oil .
Challenges and Mitigation Strategies
Racemization
The N-methyl group reduces racemization risk compared to non-methylated analogs. Maintaining pH < 9 during Boc protection further minimizes epimerization .
Byproduct Formation
-
Di-Boc Adducts : Rare due to steric hindrance from the N-methyl group.
-
Unreacted Starting Material : Eliminated via aqueous extraction .
Industrial-Scale Production
Large-scale synthesis (≥1 kg) employs continuous flow reactors to enhance mixing and heat transfer. Key parameters:
Comparative Analysis of Methods
Parameter | Laboratory-Scale | Industrial-Scale |
---|---|---|
Solvent | THF/H₂O | Ethyl acetate/H₂O |
Temperature | 20–25°C | 30°C |
Reaction Time | 17 hours | 2–4 hours |
Yield | 95–98% | 92–94% |
Purity | >98% (HPLC) | >99% (crystallized) |
Emerging Methodologies
Enzymatic Protection
Recent advances utilize lipases in non-aqueous media to catalyze Boc protection, achieving 85–90% yield with minimal racemization. This method is eco-friendly but currently limited to small-scale applications .
Microwave-Assisted Synthesis
Microwave irradiation (100 W, 70°C) reduces reaction time to 30 minutes with comparable yields (~93%). However, scalability remains a challenge .
Chemical Reactions Analysis
Types of Reactions:
Substitution Reactions: Boc-N-Me-Ala-OH can undergo nucleophilic substitution reactions where the Boc group can be replaced by other protecting groups or functional groups.
Deprotection Reactions: The Boc group can be removed under acidic conditions, typically using trifluoroacetic acid (TFA), to yield N-methyl-L-alanine.
Common Reagents and Conditions:
Nucleophilic Substitution: Reagents such as sodium hydride (NaH) or potassium carbonate (K₂CO₃) in polar aprotic solvents like dimethylformamide (DMF) are commonly used.
Deprotection: Trifluoroacetic acid (TFA) in dichloromethane (DCM) is the standard reagent for Boc deprotection.
Major Products Formed:
N-Methyl-L-Alanine: Formed after the removal of the Boc group.
Substituted Derivatives: Depending on the nucleophile used in substitution reactions.
Scientific Research Applications
Peptide Synthesis
Boc-N-Methyl-L-Alanine is primarily utilized as a building block in solid-phase peptide synthesis (SPPS). Its unique structure allows for the incorporation of N-methylated alanine residues into peptides, which can enhance their stability and reduce racemization during coupling reactions. Studies have shown that this compound significantly decreases the formation of undesired diastereomers, leading to higher yields of desired peptide products.
Table 1: Coupling Efficiency with Various Reagents
Coupling Reagent | % Racemization | % Yield |
---|---|---|
WSCI | 0.2 | 80 |
Bop | 2.7 | 95 |
HATU | 4.7 | 101 |
This table illustrates the effectiveness of different coupling reagents when used with Boc-N-Methyl-L-Alanine, showcasing its utility in achieving high yields with minimal racemization.
Biological Research
In biological studies, peptides synthesized using Boc-N-Methyl-L-Alanine are employed to investigate protein-protein interactions, enzyme-substrate interactions, and receptor-ligand binding mechanisms. The incorporation of N-methylated residues can influence the conformation and biological activity of peptides, making them valuable for studying complex biological processes.
Medicinal Chemistry
The potential therapeutic applications of peptides synthesized with Boc-N-Methyl-L-Alanine include the development of peptide-based drugs and vaccines. The stability imparted by the Boc protecting group enhances the pharmacokinetic properties of these peptides, making them more suitable for therapeutic use.
Case Studies
Recent studies have highlighted the effectiveness of using Boc-N-Methyl-L-Alanine in various applications:
- Study on Peptide Stability : Research demonstrated that peptides containing this compound exhibited enhanced stability against enzymatic degradation compared to their non-methylated counterparts.
- Anticancer Properties : Investigations into novel derivatives related to Boc-N-Methyl-L-Alanine have shown promising anticancer activities, suggesting potential applications in cancer therapeutics .
Mechanism of Action
The mechanism of action of Boc-N-Me-Ala-OH primarily involves its role as a building block in peptide synthesis. The Boc group protects the amino group during the synthesis process, preventing unwanted side reactions. Once the peptide chain is assembled, the Boc group is removed under acidic conditions to yield the final peptide product .
Comparison with Similar Compounds
Key Properties:
- CAS Number : 16948-16-6
- Molecular Formula: C₉H₁₇NO₄
- Molecular Weight : 203.24 g/mol
- Purity : ≥99.0% (TLC)
- Specific Rotation: [α]²⁰/D = −30° (c = 1% in ethanol)
- Applications : SPPS, synthesis of peptide-based therapeutics, and protease-resistant analogs .
Structural and Functional Analogues
The following table compares Boc-N-Me-Ala-OH with structurally related Boc-protected amino acids:
Key Observations:
- N-Methylation: Introduces steric hindrance, reducing hydrogen-bonding capacity and improving metabolic stability compared to non-methylated analogs like Boc-Ala-OH .
- Side Chain Variations: Boc-N-Me-Nle-OH (norleucine) enhances hydrophobicity, while Boc-N-Me-Glu(OMe)-OH adds a polar ester group for solubility modulation .
Key Observations:
- This compound achieves near-quantitative yields (95%) in peptide bond formation, outperforming Boc-N-Me-Nle-OH (82%) due to reduced steric bulk .
- The absence of N-methylation in Boc-Ala-OH marginally lowers yields (89%) in sterically demanding environments .
Diastereomer Formation and Separation
N-methylation in this compound influences stereochemical outcomes:
Key Observations:
- This compound generates equimolar diastereomers in multicomponent reactions, necessitating chromatographic separation .
- Non-methylated analogs like Boc-Ala-OH avoid this issue, simplifying purification .
Solubility and Stability
Key Observations:
Biological Activity
Boc-N-Me-Ala-OH, or N-Boc-N-methylalanine, is a derivative of the amino acid alanine that has garnered attention in peptide synthesis and biological research. This compound's unique structural features contribute to its biological activity, making it a valuable component in various applications, particularly in drug development and synthetic biology.
This compound is characterized by the presence of a tert-butoxycarbonyl (Boc) protecting group on the amino group of N-methylalanine. This modification enhances its stability and solubility in organic solvents, facilitating its use in peptide synthesis. The compound has a high purity level (≥99.0% as per TLC analysis) and is commercially available from suppliers like Sigma-Aldrich .
1. Peptide Synthesis
This compound is primarily utilized in the synthesis of peptides due to its ability to reduce racemization during coupling reactions. Studies have demonstrated that using this compound in conjunction with various coupling reagents significantly decreases the formation of undesired diastereomers, enhancing the yield of the desired peptide products .
Table 1: Coupling Efficiency of this compound
Coupling Reagent | % Racemization | % Yield |
---|---|---|
WSCI | 0.2 | 80 |
Bop | 2.7 | 95 |
HATU | 4.7 | 101 |
This table illustrates the effectiveness of different coupling reagents when used with this compound, showcasing its utility in achieving high yields with minimal racemization.
2. Cytotoxicity and Antimicrobial Activity
Research indicates that peptides containing this compound exhibit varied biological activities, including cytotoxic effects against cancer cell lines. For instance, analogs incorporating N-methylalanine have shown significant cytotoxicity against human cancer cell lines such as A549 (lung cancer) and HCT-116 (colon cancer), with IC50 values indicating potent activity .
Case Study: Cytotoxic Effects of Peptides
In a study evaluating the cytotoxic properties of synthesized peptides, those containing this compound displayed an EC50 value of approximately 10 µM against A549 cells, suggesting that this compound can effectively induce apoptosis in malignant cells .
3. Antimicrobial Properties
Additionally, peptides synthesized with this compound have demonstrated antimicrobial properties. Some studies highlight their effectiveness against various bacterial strains, suggesting potential applications in developing new antimicrobial agents .
Table 2: Antimicrobial Activity of Peptides Containing this compound
Bacterial Strain | Minimum Inhibitory Concentration (MIC) |
---|---|
E. coli | 32 µg/mL |
S. aureus | 16 µg/mL |
Pseudomonas aeruginosa | 64 µg/mL |
This table summarizes the MIC values for peptides containing this compound against selected bacterial strains, emphasizing its potential as an antimicrobial agent.
The biological activity of this compound-containing peptides is attributed to their ability to interact with cellular membranes and disrupt cellular processes. The presence of N-methyl groups may enhance hydrophobic interactions, allowing these peptides to penetrate lipid membranes more effectively .
Q & A
Basic Research Questions
Q. What are the standard protocols for synthesizing Boc-N-Me-Ala-OH, and how can purity be validated?
this compound is synthesized via Boc protection of N-methyl-L-alanine. A validated procedure involves dissolving the amino acid in water and THF, adding Boc₂O (1.1 equivalents) with NaHCO₃, and stirring overnight. After acidification (pH 3), extraction with ethyl acetate yields the product with 90% efficiency . Purity is confirmed via thin-layer chromatography (TLC) and quantitative NMR (¹H, ¹³C) to verify chemical shifts (e.g., δ 1.38 ppm for Boc methyl groups) and HRMS (ESI) for mass accuracy (e.g., m/z 226.1043 ).
Q. Which analytical techniques are critical for characterizing this compound in peptide synthesis?
Key techniques include:
- Optical rotation ([α]D = +22–32° in EtOH) to confirm stereochemical integrity .
- NMR spectroscopy to detect N-methylation (δ 2.85 ppm for -N(CH₃)) and Boc-group signals .
- HRMS to validate molecular weight (e.g., 203.24 g/mol) and isotopic patterns .
- TLC (≥99% purity) for rapid purity assessment .
Q. How should this compound be stored to prevent degradation during peptide synthesis?
Store at 2–8°C in anhydrous conditions due to its hygroscopic nature. Pre-dry the compound under vacuum before coupling reactions to avoid side reactions (e.g., racemization) .
Advanced Research Questions
Q. What experimental design considerations are critical for optimizing this compound coupling efficiency in solid-phase peptide synthesis (SPPS)?
- Coupling agents : Compare HBTU/HOBt vs. DIC/Oxyma for minimizing epimerization.
- Solvent selection : Use DMF or DCM, but avoid DMSO due to potential carbamate cleavage .
- Monitoring : Employ Kaiser tests or FT-IR to track reaction completion.
- Statistical validation : Use factorial designs to optimize molar ratios (e.g., 2–4 equivalents of this compound) .
Q. How can researchers resolve contradictions in reported optical rotation values for this compound?
Discrepancies in [α]D values (e.g., +22–32° vs. +6.8° in MeOH ) may arise from:
- Solvent polarity : Ethanol vs. methanol affects hydrogen bonding and conformation.
- Impurity profiles : Trace solvents (e.g., residual THF) or byproducts (e.g., de-Boc compounds) alter measurements.
- Method calibration : Standardize measurements using a polarimeter calibrated with sucrose or camphor sulfonic acid .
Q. What strategies mitigate the hygroscopicity of this compound in aqueous reaction systems?
- Lyophilization : Pre-lyophilize the compound to remove adsorbed water.
- Inert atmosphere : Conduct reactions under argon/N₂ to minimize moisture ingress.
- Additives : Use molecular sieves (3Å) or desiccants (MgSO₄) in non-aqueous solvents .
Q. How can computational modeling enhance the design of this compound derivatives for protease inhibition studies?
- Docking studies : Simulate interactions with protease active sites (e.g., kallikrein 7) using Schrödinger Suite or AutoDock.
- QSAR models : Correlate substituent effects (e.g., N-methylation) with inhibitory potency .
- MD simulations : Assess conformational stability of Boc-protected peptides in aqueous vs. lipid environments .
Q. Methodological and Reporting Standards
Q. What are the best practices for documenting this compound synthesis in peer-reviewed publications?
- Reproducibility : Report exact molar ratios, reaction times, and purification steps (e.g., “extracted 3× with 150 mL ethyl acetate”).
- Analytical data : Include full NMR assignments, HRMS spectra, and TLC conditions (e.g., Rf = 0.5 in 7:3 EtOAc/hexane).
- Ethical reporting : Disclose batch-specific variations (e.g., lot-to-lot purity differences) and failed attempts .
Q. How can researchers leverage "People Also Ask" (PAA) data to identify gaps in this compound literature?
- Semantic analysis : Use tools like AlsoAsked or Ahrefs to cluster PAA queries (e.g., “this compound solubility” or “racemization prevention”).
- Content gaps : Address understudied topics (e.g., solvent-free synthesis) identified via PAA trends .
Q. What frameworks (e.g., FINER, PICO) are suitable for formulating hypothesis-driven studies on this compound?
- FINER criteria : Ensure feasibility (lab resources), novelty (e.g., novel coupling methods), and relevance (e.g., peptide therapeutics).
- PICO framework :
- Population : Peptide-drug conjugates.
- Intervention : this compound incorporation.
- Comparison : Unprotected vs. Boc-protected analogs.
- Outcome : Bioavailability or proteolytic stability .
Properties
IUPAC Name |
(2S)-2-[methyl-[(2-methylpropan-2-yl)oxycarbonyl]amino]propanoic acid | |
---|---|---|
Source | PubChem | |
URL | https://pubchem.ncbi.nlm.nih.gov | |
Description | Data deposited in or computed by PubChem | |
InChI |
InChI=1S/C9H17NO4/c1-6(7(11)12)10(5)8(13)14-9(2,3)4/h6H,1-5H3,(H,11,12)/t6-/m0/s1 | |
Source | PubChem | |
URL | https://pubchem.ncbi.nlm.nih.gov | |
Description | Data deposited in or computed by PubChem | |
InChI Key |
VLHQXRIIQSTJCQ-LURJTMIESA-N | |
Source | PubChem | |
URL | https://pubchem.ncbi.nlm.nih.gov | |
Description | Data deposited in or computed by PubChem | |
Canonical SMILES |
CC(C(=O)O)N(C)C(=O)OC(C)(C)C | |
Source | PubChem | |
URL | https://pubchem.ncbi.nlm.nih.gov | |
Description | Data deposited in or computed by PubChem | |
Isomeric SMILES |
C[C@@H](C(=O)O)N(C)C(=O)OC(C)(C)C | |
Source | PubChem | |
URL | https://pubchem.ncbi.nlm.nih.gov | |
Description | Data deposited in or computed by PubChem | |
Molecular Formula |
C9H17NO4 | |
Source | PubChem | |
URL | https://pubchem.ncbi.nlm.nih.gov | |
Description | Data deposited in or computed by PubChem | |
Molecular Weight |
203.24 g/mol | |
Source | PubChem | |
URL | https://pubchem.ncbi.nlm.nih.gov | |
Description | Data deposited in or computed by PubChem | |
CAS No. |
16948-16-6 | |
Record name | (2S)-2-{[(tert-butoxy)carbonyl](methyl)amino}propanoic acid | |
Source | European Chemicals Agency (ECHA) | |
URL | https://echa.europa.eu/information-on-chemicals | |
Description | The European Chemicals Agency (ECHA) is an agency of the European Union which is the driving force among regulatory authorities in implementing the EU's groundbreaking chemicals legislation for the benefit of human health and the environment as well as for innovation and competitiveness. | |
Explanation | Use of the information, documents and data from the ECHA website is subject to the terms and conditions of this Legal Notice, and subject to other binding limitations provided for under applicable law, the information, documents and data made available on the ECHA website may be reproduced, distributed and/or used, totally or in part, for non-commercial purposes provided that ECHA is acknowledged as the source: "Source: European Chemicals Agency, http://echa.europa.eu/". Such acknowledgement must be included in each copy of the material. ECHA permits and encourages organisations and individuals to create links to the ECHA website under the following cumulative conditions: Links can only be made to webpages that provide a link to the Legal Notice page. | |
Retrosynthesis Analysis
AI-Powered Synthesis Planning: Our tool employs the Template_relevance Pistachio, Template_relevance Bkms_metabolic, Template_relevance Pistachio_ringbreaker, Template_relevance Reaxys, Template_relevance Reaxys_biocatalysis model, leveraging a vast database of chemical reactions to predict feasible synthetic routes.
One-Step Synthesis Focus: Specifically designed for one-step synthesis, it provides concise and direct routes for your target compounds, streamlining the synthesis process.
Accurate Predictions: Utilizing the extensive PISTACHIO, BKMS_METABOLIC, PISTACHIO_RINGBREAKER, REAXYS, REAXYS_BIOCATALYSIS database, our tool offers high-accuracy predictions, reflecting the latest in chemical research and data.
Strategy Settings
Precursor scoring | Relevance Heuristic |
---|---|
Min. plausibility | 0.01 |
Model | Template_relevance |
Template Set | Pistachio/Bkms_metabolic/Pistachio_ringbreaker/Reaxys/Reaxys_biocatalysis |
Top-N result to add to graph | 6 |
Feasible Synthetic Routes
Disclaimer and Information on In-Vitro Research Products
Please be aware that all articles and product information presented on BenchChem are intended solely for informational purposes. The products available for purchase on BenchChem are specifically designed for in-vitro studies, which are conducted outside of living organisms. In-vitro studies, derived from the Latin term "in glass," involve experiments performed in controlled laboratory settings using cells or tissues. It is important to note that these products are not categorized as medicines or drugs, and they have not received approval from the FDA for the prevention, treatment, or cure of any medical condition, ailment, or disease. We must emphasize that any form of bodily introduction of these products into humans or animals is strictly prohibited by law. It is essential to adhere to these guidelines to ensure compliance with legal and ethical standards in research and experimentation.