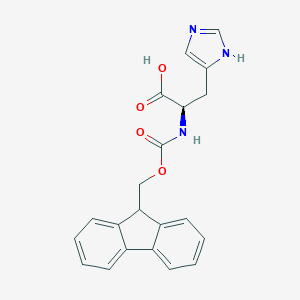
FMOC-D-HIS-OH
Overview
Description
FMOC-D-HIS-OH: , also known as Nα-Fmoc-D-histidine, is a derivative of the amino acid histidine. It is widely used in peptide synthesis due to its ability to protect the amino group during the synthesis process. The FMOC group (fluorenylmethyloxycarbonyl) is a base-labile protecting group that can be easily removed under mild conditions, making it a popular choice in solid-phase peptide synthesis.
Mechanism of Action
Target of Action
The primary target of Fmoc-D-His is the amine group of amino acids . The Fmoc group is frequently used as a protecting group for amines, where it can be introduced by reacting the amine with fluorenylmethyloxycarbonyl chloride (Fmoc-Cl) .
Mode of Action
The Fmoc group acts as a protecting group for the amine group of amino acids during peptide synthesis . It is introduced by reacting the amine with Fmoc-Cl . The Fmoc group is base-labile, meaning it can be removed under basic conditions . Piperidine is usually preferred for Fmoc group removal as it forms a stable adduct with the dibenzofulvene byproduct, preventing it from reacting with the substrate .
Biochemical Pathways
The Fmoc group plays a crucial role in the chemical synthesis of peptides . It allows for the rapid and highly efficient synthesis of peptides, including ones of significant size and complexity .
Pharmacokinetics
The fmoc group’s stability to treatment with trifluoroacetic acid (tfa) and hydrogen bromide/acetic acid has been noted . This stability suggests that the Fmoc group may influence the ADME (Absorption, Distribution, Metabolism, and Excretion) properties of the compound.
Result of Action
The primary result of Fmoc-D-His’s action is the formation of protected peptides during peptide synthesis . The Fmoc group protects the amine group of amino acids, allowing for the efficient synthesis of complex peptides .
Action Environment
The action of Fmoc-D-His is influenced by the pH of the environment . The Fmoc group is base-labile, meaning it can be removed under basic conditions . Therefore, the pH of the environment can influence the efficacy and stability of Fmoc-D-His. Additionally, the Fmoc group is stable to treatment with TFA and hydrogen bromide/acetic acid, suggesting that it may also be stable under acidic conditions .
Biochemical Analysis
Biochemical Properties
In biochemical reactions, FMOC-D-HIS-OH plays a crucial role as a building block in peptide synthesis . The Fmoc group in this compound can be introduced by reacting the amine with fluorenylmethyloxycarbonyl chloride (Fmoc-Cl) . This process is essential in the formation of peptides, as it provides protection against racemization and by-product formation .
Cellular Effects
The cellular effects of this compound are primarily related to its role in peptide synthesis. As a building block in this process, this compound contributes to the formation of peptides that can influence various cellular processes, including cell signaling pathways, gene expression, and cellular metabolism .
Molecular Mechanism
The molecular mechanism of this compound involves its role as a protecting group in peptide synthesis. The Fmoc group in this compound is base-labile, meaning it can be rapidly removed by a base . This property allows for the controlled assembly and disassembly of peptides during synthesis .
Temporal Effects in Laboratory Settings
In laboratory settings, the effects of this compound can change over time, particularly in relation to its stability and degradation. The Fmoc group in this compound is stable under certain conditions, which is crucial for its role in peptide synthesis . It can be rapidly removed by a base, such as piperidine .
Metabolic Pathways
This compound is involved in the metabolic pathway of peptide synthesis . In this process, this compound interacts with other amino acids, enzymes, and cofactors to form peptides .
Transport and Distribution
The transport and distribution of this compound within cells and tissues are closely tied to its role in peptide synthesis. As a building block in this process, this compound is transported to the site of peptide synthesis where it interacts with other molecules to form peptides .
Subcellular Localization
The subcellular localization of this compound is related to its role in peptide synthesis. During this process, this compound is typically localized at the site of peptide synthesis . The exact subcellular localization can vary depending on the specific cellular context and the other molecules involved in the synthesis process.
Preparation Methods
Synthetic Routes and Reaction Conditions:
Alternative Methods: Another method involves using 9-fluorenylmethylsuccinimidyl carbonate (FMOC-OSu), which can be obtained by reacting FMOC-Cl with the dicyclohexylammonium salt of N-hydroxysuccinimide.
Industrial Production Methods: The industrial production of FMOC-D-HIS-OH typically follows the same synthetic routes as described above but on a larger scale. The reaction conditions are optimized to ensure high yield and purity of the product.
Chemical Reactions Analysis
Types of Reactions:
Substitution Reactions: FMOC-D-HIS-OH can undergo substitution reactions where the FMOC group is replaced by another protecting group or functional group.
Deprotection Reactions: The FMOC group can be removed by treatment with a base such as piperidine in N,N-dimethylformamide (DMF), resulting in the formation of the free amino group.
Common Reagents and Conditions:
FMOC Protection: FMOC-Cl, sodium bicarbonate, aqueous dioxane.
Deprotection: Piperidine, DMF.
Major Products:
Protected Amino Acid: this compound.
Deprotected Amino Acid: D-histidine.
Scientific Research Applications
Peptide Synthesis
Role in Peptide Synthesis:
FMOC-D-HIS-OH is primarily utilized as a protective group in solid-phase peptide synthesis (SPPS). Its stability under various conditions allows for selective modification of amino acids, which is vital for constructing complex peptides with specific sequences.
Advantages:
- Reduces unwanted side reactions during peptide assembly.
- Facilitates the incorporation of histidine residues into peptides, which are important for biological activity.
Drug Development
Application in Pharmaceuticals:
The compound aids in the design of novel pharmaceuticals by enhancing the targeting of specific biological pathways. This is particularly significant in developing peptide-based drugs that require precise interactions with receptors or enzymes.
Case Study:
A study highlighted the use of this compound in developing peptide drugs that target cancer cells more effectively than traditional therapies. By modifying the peptide structure using this compound, researchers achieved improved binding affinity to cancer-specific receptors, resulting in enhanced therapeutic efficacy .
Bioconjugation
Bioconjugation Processes:
this compound is instrumental in bioconjugation, where it facilitates the attachment of biomolecules to surfaces or other molecules. This property is crucial for developing biosensors and targeted drug delivery systems.
Research Insight:
In a recent study, this compound was used to conjugate peptides to nanoparticles for targeted delivery in cancer therapy. The conjugation improved the specificity and efficacy of drug delivery systems, demonstrating its potential in clinical applications .
Research in Cancer Therapy
Targeted Therapies:
The synthesis of histidine-containing peptides using this compound has shown promise in cancer research. These peptides can be tailored to improve treatment outcomes by targeting specific tumor markers.
Case Study:
Research demonstrated that peptides synthesized with this compound exhibited enhanced cytotoxicity against cancer cell lines compared to those synthesized with other histidine derivatives. This finding underscores the importance of histidine's role in peptide functionality and therapeutic potential .
Protein Engineering
Modification of Proteins:
this compound is crucial for protein engineering, allowing scientists to create modified proteins with enhanced properties for various applications, including enzyme design and therapeutic proteins.
Example:
In a study focused on enzyme engineering, researchers utilized this compound to incorporate histidine residues into enzyme structures, thereby improving their catalytic efficiency and stability under physiological conditions .
Data Table: Applications Overview
Application Area | Description | Key Benefits |
---|---|---|
Peptide Synthesis | Used as a protective group in SPPS | Prevents side reactions; enables complex peptides |
Drug Development | Aids in designing peptide-based drugs targeting specific pathways | Enhances binding affinity; improves efficacy |
Bioconjugation | Facilitates attachment of biomolecules for biosensors and drug delivery systems | Improves specificity; enhances delivery capabilities |
Cancer Therapy | Used to synthesize targeted therapies containing histidine | Increases cytotoxicity against cancer cells |
Protein Engineering | Modifies proteins to enhance stability and activity | Improves catalytic efficiency |
Comparison with Similar Compounds
FMOC-L-HIS-OH: The L-enantiomer of FMOC-D-HIS-OH, used in similar applications but with different stereochemistry.
FMOC-TRP-OH: FMOC-protected tryptophan, another amino acid used in peptide synthesis.
FMOC-ARG-OH: FMOC-protected arginine, used in the synthesis of peptides containing arginine residues.
Uniqueness: this compound is unique due to its D-configuration, which can impart different biological properties compared to its L-counterpart. This makes it valuable in the synthesis of peptides with specific stereochemical requirements .
Biological Activity
FMOC-D-HIS-OH, or Fmoc-D-histidine, is a derivative of the amino acid histidine, which plays a critical role in various biological processes. This article explores the biological activity of this compound, focusing on its synthesis, stability, and implications in peptide synthesis and pharmacology.
1. Overview of this compound
This compound is an Nα-Fmoc-protected form of D-histidine, commonly used in solid-phase peptide synthesis (SPPS). The Fmoc (9-fluorenylmethyloxycarbonyl) group serves as a protective group that facilitates the coupling of amino acids while preventing unwanted reactions. The D-isomer of histidine is particularly significant due to its unique biological properties compared to the L-isomer.
2. Synthesis and Stability
The synthesis of this compound typically involves protecting the amino group with the Fmoc group and ensuring minimal racemization during the coupling process. Histidine is known for its susceptibility to epimerization, which can alter its biological activity. Studies have shown that careful control of reaction conditions can mitigate racemization rates:
- Epimerization Risk : The lone pair electrons on the imidazole nitrogen can lead to intramolecular side reactions, increasing the likelihood of forming D-histidine from L-histidine during activation in SPPS .
- Stability Studies : Research indicates that different derivatives of histidine exhibit varying stability under similar conditions. For instance, Fmoc-His(Boc)-OH demonstrated greater stability than Fmoc-His(Trt)-OH, which showed significant discoloration and impurity formation over time .
3. Biological Activity
The biological activity of this compound is primarily influenced by its structural properties and how it interacts with biological systems:
- Peptide Formation : this compound is utilized in synthesizing peptides that can modulate biological functions, including receptor binding and enzyme activity. Its incorporation into peptides can enhance their stability and efficacy .
- Binding Affinity : In studies involving cyclic peptides with D-histidine, researchers found that modifications at specific positions could significantly improve binding affinities to human melanocortin receptors (hMC1R, hMC4R). For instance, certain analogues exhibited IC50 values as low as 0.7 nM for hMC4R, indicating strong receptor interactions .
4.1 Peptide Synthesis and Evaluation
A study evaluated the incorporation of this compound into peptide sequences aimed at targeting specific receptors related to obesity and sexual dysfunction:
- Peptide Design : Novel cyclic analogues were synthesized using this compound, demonstrating enhanced selectivity and potency for melanocortin receptors.
- Biological Assays : Binding assays revealed that peptides containing D-histidine had improved receptor selectivity compared to their L-counterparts, suggesting a potential therapeutic application in metabolic disorders .
4.2 Stability and Racemization Analysis
Research conducted on the stability of various histidine derivatives highlighted the importance of protecting groups in maintaining enantiomeric purity:
- Racemization Rates : The study reported racemization rates for TBTU-assisted coupling reactions involving this compound, emphasizing that optimal conditions can minimize unwanted side reactions .
- Purity Assessment : UPLC analysis confirmed that under controlled conditions, this compound maintained over 99% purity throughout the synthesis process .
5. Conclusion
This compound serves as a valuable tool in peptide synthesis due to its unique properties and biological relevance. Its ability to enhance peptide stability and binding affinity makes it an important compound for research in pharmacology and biochemistry. Ongoing studies are likely to further elucidate its role in therapeutic applications.
6. Data Tables
Property | Value |
---|---|
Chemical Name | This compound |
Molecular Weight | 295.34 g/mol |
Purity | >99% |
Typical IC50 (hMC4R) | 0.7 nM |
Stability (in DMF) | Colorless after 10 days |
7. References
Properties
IUPAC Name |
(2R)-2-(9H-fluoren-9-ylmethoxycarbonylamino)-3-(1H-imidazol-5-yl)propanoic acid | |
---|---|---|
Source | PubChem | |
URL | https://pubchem.ncbi.nlm.nih.gov | |
Description | Data deposited in or computed by PubChem | |
InChI |
InChI=1S/C21H19N3O4/c25-20(26)19(9-13-10-22-12-23-13)24-21(27)28-11-18-16-7-3-1-5-14(16)15-6-2-4-8-17(15)18/h1-8,10,12,18-19H,9,11H2,(H,22,23)(H,24,27)(H,25,26)/t19-/m1/s1 | |
Source | PubChem | |
URL | https://pubchem.ncbi.nlm.nih.gov | |
Description | Data deposited in or computed by PubChem | |
InChI Key |
SIRPVCUJLVXZPW-LJQANCHMSA-N | |
Source | PubChem | |
URL | https://pubchem.ncbi.nlm.nih.gov | |
Description | Data deposited in or computed by PubChem | |
Canonical SMILES |
C1=CC=C2C(=C1)C(C3=CC=CC=C32)COC(=O)NC(CC4=CN=CN4)C(=O)O | |
Source | PubChem | |
URL | https://pubchem.ncbi.nlm.nih.gov | |
Description | Data deposited in or computed by PubChem | |
Isomeric SMILES |
C1=CC=C2C(=C1)C(C3=CC=CC=C32)COC(=O)N[C@H](CC4=CN=CN4)C(=O)O | |
Source | PubChem | |
URL | https://pubchem.ncbi.nlm.nih.gov | |
Description | Data deposited in or computed by PubChem | |
Molecular Formula |
C21H19N3O4 | |
Source | PubChem | |
URL | https://pubchem.ncbi.nlm.nih.gov | |
Description | Data deposited in or computed by PubChem | |
DSSTOX Substance ID |
DTXSID50427162 | |
Record name | Nalpha-Fmoc-D-histidine | |
Source | EPA DSSTox | |
URL | https://comptox.epa.gov/dashboard/DTXSID50427162 | |
Description | DSSTox provides a high quality public chemistry resource for supporting improved predictive toxicology. | |
Molecular Weight |
377.4 g/mol | |
Source | PubChem | |
URL | https://pubchem.ncbi.nlm.nih.gov | |
Description | Data deposited in or computed by PubChem | |
CAS No. |
157355-79-8 | |
Record name | Nalpha-Fmoc-D-histidine | |
Source | EPA DSSTox | |
URL | https://comptox.epa.gov/dashboard/DTXSID50427162 | |
Description | DSSTox provides a high quality public chemistry resource for supporting improved predictive toxicology. | |
Disclaimer and Information on In-Vitro Research Products
Please be aware that all articles and product information presented on BenchChem are intended solely for informational purposes. The products available for purchase on BenchChem are specifically designed for in-vitro studies, which are conducted outside of living organisms. In-vitro studies, derived from the Latin term "in glass," involve experiments performed in controlled laboratory settings using cells or tissues. It is important to note that these products are not categorized as medicines or drugs, and they have not received approval from the FDA for the prevention, treatment, or cure of any medical condition, ailment, or disease. We must emphasize that any form of bodily introduction of these products into humans or animals is strictly prohibited by law. It is essential to adhere to these guidelines to ensure compliance with legal and ethical standards in research and experimentation.