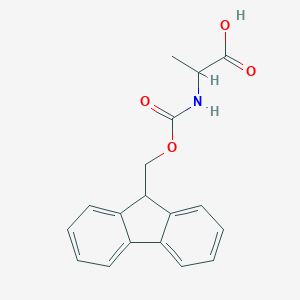
Fmoc-Ala-OH
Overview
Description
Fmoc-L-Alanine, also known as N-(9-Fluorenylmethoxycarbonyl)-L-alanine, is a derivative of the amino acid alanine. It is widely used in the field of peptide synthesis, particularly in the Fmoc solid-phase peptide synthesis method. The Fmoc group serves as a protecting group for the amino group of alanine, allowing for selective reactions to occur at other functional groups without interference.
Mechanism of Action
Target of Action
Fmoc-Ala-OH, also known as Fmoc-L-alanine, is primarily used in the field of peptide synthesis . Its main target is the N-terminal amino group of the amino acid in the peptide chain .
Mode of Action
This compound functions as a protecting group for the N-terminal amino group of the amino acid, allowing for selective deprotection and subsequent coupling reactions . It interacts at the molecular level by forming stable amide bonds with the carboxyl group of the C-terminal amino acid, enabling the controlled and sequential addition of amino acids to the growing peptide chain .
Biochemical Pathways
This compound plays a pivotal role in solid-phase peptide synthesis (SPPS), a process that facilitates the stepwise elongation of peptide chains . It is commonly used as a building block in the preparation of triazolopeptides and azapeptides . It is also used in the synthesis of bis-cationic porphyrin peptides using the standard Fmoc solid-phase synthesis .
Result of Action
The primary result of this compound’s action is the successful and efficient synthesis of peptides. By temporarily masking the N-terminal amino group, it prevents unwanted side reactions during the peptide assembly process . This leads to the formation of peptide chains with the desired sequence and length.
Action Environment
The action of this compound is influenced by various environmental factors such as temperature, pH, and the presence of other reagents. For instance, the Fmoc group is rapidly removed by base . Therefore, the conditions under which peptide synthesis is carried out can significantly impact the efficiency and success of the process.
Biochemical Analysis
Biochemical Properties
Fmoc-Ala-OH interacts with various biomolecules in biochemical reactions. It serves as a building block in the preparation of peptides, where it forms stable amide bonds with the carboxyl group of the C-terminal amino acid . This enables the controlled and sequential addition of amino acids to the growing peptide chain
Cellular Effects
The effects of this compound on cells and cellular processes are complex and multifaceted. For instance, in the context of perovskite solar cells, this compound has been shown to improve the quality of the perovskite surface, thereby enhancing device performance
Molecular Mechanism
The molecular mechanism of this compound primarily involves its role in peptide synthesis. It forms stable amide bonds with the carboxyl group of the C-terminal amino acid, enabling the controlled and sequential addition of amino acids to the growing peptide chain This process may involve binding interactions with biomolecules, enzyme inhibition or activation, and changes in gene expression
Temporal Effects in Laboratory Settings
It is known that this compound is a stable compound used in peptide synthesis
Preparation Methods
Synthetic Routes and Reaction Conditions: Fmoc-L-Alanine can be synthesized through the reaction of L-alanine with 9-fluorenylmethoxycarbonyl chloride (Fmoc-Cl) in the presence of a base such as sodium bicarbonate. The reaction typically occurs in an organic solvent like dioxane or dimethylformamide (DMF) at room temperature. The Fmoc group is introduced to the amino group of alanine, forming Fmoc-L-Alanine .
Industrial Production Methods: Industrial production of Fmoc-L-Alanine follows similar synthetic routes but on a larger scale. The process involves the use of automated peptide synthesizers that can handle large quantities of reagents and solvents. The reaction conditions are optimized to ensure high yield and purity of the final product. The product is then purified using techniques such as crystallization or chromatography .
Chemical Reactions Analysis
Types of Reactions: Fmoc-L-Alanine undergoes various chemical reactions, including:
Deprotection: The Fmoc group can be removed using a base such as piperidine in DMF, yielding free L-alanine.
Coupling Reactions: It can participate in peptide bond formation with other amino acids or peptides using coupling reagents like HBTU or DIC.
Common Reagents and Conditions:
Deprotection: 20% piperidine in DMF is commonly used for Fmoc deprotection.
Major Products Formed:
Deprotection: Free L-alanine.
Coupling: Peptides or peptide fragments with Fmoc-L-Alanine incorporated into the sequence.
Scientific Research Applications
Fmoc-L-Alanine is extensively used in scientific research, particularly in the following areas:
Comparison with Similar Compounds
Fmoc-L-Phenylalanine: Similar to Fmoc-L-Alanine but with a phenyl group as the side chain.
Fmoc-L-Valine: Contains a valine side chain instead of alanine.
Fmoc-L-Leucine: Features a leucine side chain.
Uniqueness: Fmoc-L-Alanine is unique due to its small side chain, which confers a high degree of flexibility when incorporated into peptides. This flexibility is advantageous in the synthesis of peptides with complex structures and functions .
Properties
IUPAC Name |
(2S)-2-(9H-fluoren-9-ylmethoxycarbonylamino)propanoic acid | |
---|---|---|
Source | PubChem | |
URL | https://pubchem.ncbi.nlm.nih.gov | |
Description | Data deposited in or computed by PubChem | |
InChI |
InChI=1S/C18H17NO4/c1-11(17(20)21)19-18(22)23-10-16-14-8-4-2-6-12(14)13-7-3-5-9-15(13)16/h2-9,11,16H,10H2,1H3,(H,19,22)(H,20,21)/t11-/m0/s1 | |
Source | PubChem | |
URL | https://pubchem.ncbi.nlm.nih.gov | |
Description | Data deposited in or computed by PubChem | |
InChI Key |
QWXZOFZKSQXPDC-NSHDSACASA-N | |
Source | PubChem | |
URL | https://pubchem.ncbi.nlm.nih.gov | |
Description | Data deposited in or computed by PubChem | |
Canonical SMILES |
CC(C(=O)O)NC(=O)OCC1C2=CC=CC=C2C3=CC=CC=C13 | |
Source | PubChem | |
URL | https://pubchem.ncbi.nlm.nih.gov | |
Description | Data deposited in or computed by PubChem | |
Isomeric SMILES |
C[C@@H](C(=O)O)NC(=O)OCC1C2=CC=CC=C2C3=CC=CC=C13 | |
Source | PubChem | |
URL | https://pubchem.ncbi.nlm.nih.gov | |
Description | Data deposited in or computed by PubChem | |
Molecular Formula |
C18H17NO4 | |
Source | PubChem | |
URL | https://pubchem.ncbi.nlm.nih.gov | |
Description | Data deposited in or computed by PubChem | |
DSSTOX Substance ID |
DTXSID001245823 | |
Record name | N-[(9H-Fluoren-9-ylmethoxy)carbonyl]-L-alanine | |
Source | EPA DSSTox | |
URL | https://comptox.epa.gov/dashboard/DTXSID001245823 | |
Description | DSSTox provides a high quality public chemistry resource for supporting improved predictive toxicology. | |
Molecular Weight |
311.3 g/mol | |
Source | PubChem | |
URL | https://pubchem.ncbi.nlm.nih.gov | |
Description | Data deposited in or computed by PubChem | |
Physical Description |
White to light yellow solid; [EMD Millipore MSDS] | |
Record name | N-((9H-Fluoren-9-ylmethoxy)carbonyl)-L-alanine | |
Source | Haz-Map, Information on Hazardous Chemicals and Occupational Diseases | |
URL | https://haz-map.com/Agents/20866 | |
Description | Haz-Map® is an occupational health database designed for health and safety professionals and for consumers seeking information about the adverse effects of workplace exposures to chemical and biological agents. | |
Explanation | Copyright (c) 2022 Haz-Map(R). All rights reserved. Unless otherwise indicated, all materials from Haz-Map are copyrighted by Haz-Map(R). No part of these materials, either text or image may be used for any purpose other than for personal use. Therefore, reproduction, modification, storage in a retrieval system or retransmission, in any form or by any means, electronic, mechanical or otherwise, for reasons other than personal use, is strictly prohibited without prior written permission. | |
CAS No. |
35661-39-3 | |
Record name | N-[(9H-Fluoren-9-ylmethoxy)carbonyl]-L-alanine | |
Source | CAS Common Chemistry | |
URL | https://commonchemistry.cas.org/detail?cas_rn=35661-39-3 | |
Description | CAS Common Chemistry is an open community resource for accessing chemical information. Nearly 500,000 chemical substances from CAS REGISTRY cover areas of community interest, including common and frequently regulated chemicals, and those relevant to high school and undergraduate chemistry classes. This chemical information, curated by our expert scientists, is provided in alignment with our mission as a division of the American Chemical Society. | |
Explanation | The data from CAS Common Chemistry is provided under a CC-BY-NC 4.0 license, unless otherwise stated. | |
Record name | N-[(9H-Fluoren-9-ylmethoxy)carbonyl]-L-alanine | |
Source | EPA DSSTox | |
URL | https://comptox.epa.gov/dashboard/DTXSID001245823 | |
Description | DSSTox provides a high quality public chemistry resource for supporting improved predictive toxicology. | |
Record name | N-[(9H-fluoren-9-ylmethoxy)carbonyl]-L-alanine | |
Source | European Chemicals Agency (ECHA) | |
URL | https://echa.europa.eu/substance-information/-/substanceinfo/100.047.858 | |
Description | The European Chemicals Agency (ECHA) is an agency of the European Union which is the driving force among regulatory authorities in implementing the EU's groundbreaking chemicals legislation for the benefit of human health and the environment as well as for innovation and competitiveness. | |
Explanation | Use of the information, documents and data from the ECHA website is subject to the terms and conditions of this Legal Notice, and subject to other binding limitations provided for under applicable law, the information, documents and data made available on the ECHA website may be reproduced, distributed and/or used, totally or in part, for non-commercial purposes provided that ECHA is acknowledged as the source: "Source: European Chemicals Agency, http://echa.europa.eu/". Such acknowledgement must be included in each copy of the material. ECHA permits and encourages organisations and individuals to create links to the ECHA website under the following cumulative conditions: Links can only be made to webpages that provide a link to the Legal Notice page. | |
Synthesis routes and methods
Procedure details
Retrosynthesis Analysis
AI-Powered Synthesis Planning: Our tool employs the Template_relevance Pistachio, Template_relevance Bkms_metabolic, Template_relevance Pistachio_ringbreaker, Template_relevance Reaxys, Template_relevance Reaxys_biocatalysis model, leveraging a vast database of chemical reactions to predict feasible synthetic routes.
One-Step Synthesis Focus: Specifically designed for one-step synthesis, it provides concise and direct routes for your target compounds, streamlining the synthesis process.
Accurate Predictions: Utilizing the extensive PISTACHIO, BKMS_METABOLIC, PISTACHIO_RINGBREAKER, REAXYS, REAXYS_BIOCATALYSIS database, our tool offers high-accuracy predictions, reflecting the latest in chemical research and data.
Strategy Settings
Precursor scoring | Relevance Heuristic |
---|---|
Min. plausibility | 0.01 |
Model | Template_relevance |
Template Set | Pistachio/Bkms_metabolic/Pistachio_ringbreaker/Reaxys/Reaxys_biocatalysis |
Top-N result to add to graph | 6 |
Feasible Synthetic Routes
Q1: What are the primary applications of Fmoc-Ala-OH in scientific research?
A1: this compound serves as a key building block in peptide synthesis. Its primary application lies in solid-phase peptide synthesis, where it facilitates the step-by-step addition of alanine residues to a growing peptide chain [, ]. This process is crucial for creating peptides for various research applications, including drug discovery and development.
Q2: Can this compound self-assemble, and if so, what structures does it form?
A2: Yes, this compound demonstrates self-assembling properties []. Studies have shown that it forms flower-like structures both at low and high concentrations under room temperature and upon heating to 70°C []. This self-assembly behavior is attributed to various intermolecular interactions, including hydrogen bonding and π-π stacking between the Fmoc groups.
Q3: Have any impurities been identified in commercially available this compound, and what are their implications?
A3: Research has identified Fmoc-β-Ala-Ala-OH as an impurity in this compound, leading to the unexpected formation of β-alanine insertion mutants during peptide synthesis [, ]. This contamination highlights the need for stringent quality control measures in Fmoc-amino acid production to ensure the accuracy and reliability of peptide synthesis.
Q4: How does the structure of this compound relate to its function in peptide synthesis?
A4: The Fmoc group in this compound acts as a temporary protecting group for the amino group of alanine. This protection is crucial during peptide synthesis, preventing unwanted side reactions and ensuring the controlled addition of amino acids to the growing peptide chain. The Fmoc group can be selectively removed under mild basic conditions, allowing for further amino acid coupling.
Q5: Are there any studies on using this compound in material science applications?
A5: While primarily known for its role in peptide synthesis, research has explored the use of this compound in material science. For instance, one study investigated its potential in perovskite solar cells, focusing on its ability to passivate the interface and enhance device performance []. This exploration suggests this compound's potential applications beyond traditional peptide chemistry.
Q6: Can this compound be incorporated into cyclic peptides, and if so, what advantages does this offer?
A6: Yes, this compound can be incorporated into cyclic peptides []. This incorporation can enhance the peptide's stability against enzymatic degradation and improve its binding affinity and selectivity towards target molecules. This property makes cyclic peptides containing this compound attractive candidates for developing therapeutic agents and diagnostic tools.
Q7: Has this compound been used in the synthesis of any bioactive peptides?
A7: Researchers have utilized this compound in synthesizing various bioactive peptides, including a novel analog of endomorphin-2 []. This analog, H-Tyr-cAsn(acetone)-Phe-Phe-NH2, demonstrated antinociceptive activity in mice, highlighting the potential of this compound in developing novel therapeutic peptides.
Disclaimer and Information on In-Vitro Research Products
Please be aware that all articles and product information presented on BenchChem are intended solely for informational purposes. The products available for purchase on BenchChem are specifically designed for in-vitro studies, which are conducted outside of living organisms. In-vitro studies, derived from the Latin term "in glass," involve experiments performed in controlled laboratory settings using cells or tissues. It is important to note that these products are not categorized as medicines or drugs, and they have not received approval from the FDA for the prevention, treatment, or cure of any medical condition, ailment, or disease. We must emphasize that any form of bodily introduction of these products into humans or animals is strictly prohibited by law. It is essential to adhere to these guidelines to ensure compliance with legal and ethical standards in research and experimentation.