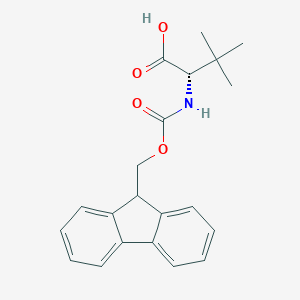
Fmoc-Tle-OH
Overview
Description
Fmoc-Tle-OH: N-(9-fluorenylmethoxycarbonyl)-L-tert-leucine , is a derivative of the amino acid leucine. It is characterized by the presence of a fluorenylmethoxycarbonyl (Fmoc) protecting group, which is commonly used in peptide synthesis. This compound appears as a white to light yellow crystalline powder and is soluble in organic solvents like dimethyl sulfoxide (DMSO) and dichloromethane (DCM), but insoluble in water .
Mechanism of Action
Target of Action
Fmoc-Tle-OH, also known as Fmoc-L-tert-leucine or Fmoc-tBu-Gly-OH, is a derivative of the amino acid leucine . As an amino acid derivative, it primarily targets the protein synthesis machinery in cells, specifically the ribosomes that are responsible for peptide chain elongation .
Mode of Action
This compound is used in solid-phase peptide synthesis (SPPS) as a building block . The Fmoc (9-fluorenylmethyloxycarbonyl) group serves as a temporary protecting group for the amino group during peptide synthesis . It is removed by a base, usually piperidine, which forms a stable adduct with the dibenzofulvene byproduct, preventing it from reacting with the substrate .
Biochemical Pathways
The primary biochemical pathway influenced by this compound is protein synthesis. Specifically, it is involved in the elongation step of peptide chain formation during translation . The Fmoc group’s removal allows the amino acid to be incorporated into the growing peptide chain .
Result of Action
The primary result of this compound’s action is the synthesis of peptides. By serving as a building block in peptide synthesis, it contributes to the formation of proteins, which are crucial for numerous biological functions .
Action Environment
The action of this compound is influenced by various environmental factors. For instance, its stability can be affected by light and temperature . It is recommended to store this compound in a dry, cool place, away from light . Furthermore, the efficiency of Fmoc group removal can be influenced by the choice of base and reaction conditions .
Biochemical Analysis
Biochemical Properties
Fmoc-L-tert-leucine plays a significant role in biochemical reactions, particularly in the synthesis of peptides . It interacts with various biomolecules during these processes. For instance, it reacts with the amine group of an amino acid during peptide bond formation . The nature of these interactions is largely determined by the chemical properties of Fmoc-L-tert-leucine, including its reactivity and stability under different conditions .
Cellular Effects
The effects of Fmoc-L-tert-leucine on cells are primarily related to its role in peptide synthesis. It influences cell function by contributing to the production of peptides, which can have various roles in cellular processes, including cell signaling pathways, gene expression, and cellular metabolism . The specific effects can vary depending on the nature of the peptides being synthesized.
Molecular Mechanism
The molecular mechanism of action of Fmoc-L-tert-leucine involves its role as a protecting group in peptide synthesis . It protects the amine group of an amino acid from reacting prematurely during the synthesis process . Once the peptide bond is formed, the Fmoc group can be removed under basic conditions, allowing the peptide chain to continue growing .
Temporal Effects in Laboratory Settings
In laboratory settings, the effects of Fmoc-L-tert-leucine can change over time, particularly during the course of a peptide synthesis reaction . The compound is stable under the conditions used for peptide synthesis, but it can be removed when the conditions are made basic . This property is crucial for its role in peptide synthesis, as it allows for the controlled growth of the peptide chain .
Metabolic Pathways
Fmoc-L-tert-leucine is involved in the metabolic pathway of peptide synthesis During this process, it interacts with various enzymes and other biomolecules
Preparation Methods
Synthetic Routes and Reaction Conditions: The preparation of Fmoc-Tle-OH typically involves the following steps:
Synthesis of tert-leucine precursor: The initial step involves synthesizing the tert-leucine precursor using appropriate starting materials.
Introduction of the Fmoc group: The tert-leucine precursor is then reacted with fluorenylmethoxycarbonyl chloride (Fmoc-Cl) to introduce the Fmoc protecting group.
Purification and Crystallization: The final product is purified and crystallized using suitable solvents to obtain this compound in high purity.
Industrial Production Methods: Industrial production of this compound follows similar synthetic routes but on a larger scale. The process involves optimizing reaction conditions to ensure high yield and purity. The use of automated systems and advanced purification techniques like high-performance liquid chromatography (HPLC) is common in industrial settings .
Chemical Reactions Analysis
Types of Reactions: Fmoc-Tle-OH undergoes various chemical reactions, including:
Substitution Reactions: The Fmoc group can be introduced or removed through substitution reactions.
Coupling Reactions: this compound is commonly used in peptide synthesis, where it undergoes coupling reactions with other amino acids to form peptide bonds.
Common Reagents and Conditions:
Fmoc-Cl: Used to introduce the Fmoc group.
Piperidine: Used for the removal of the Fmoc group during peptide synthesis.
Dicyclohexylcarbodiimide (DCC): Used as a coupling reagent in peptide synthesis.
Major Products Formed:
Scientific Research Applications
Chemistry:
Peptide Synthesis: Fmoc-Tle-OH is widely used in solid-phase peptide synthesis (SPPS) to protect the amino group of amino acids during the synthesis process.
Biology:
Protein Engineering: It is used in the synthesis of peptides and proteins with specific sequences for studying protein structure and function.
Medicine:
Drug Development: this compound is used in the synthesis of peptide-based drugs and therapeutic agents.
Industry:
Comparison with Similar Compounds
Fmoc-Leu-OH: Similar to Fmoc-Tle-OH but derived from leucine instead of tert-leucine.
Fmoc-Gly-OH: Derived from glycine and used in peptide synthesis.
Fmoc-Pro-OH: Derived from proline and used in peptide synthesis.
Uniqueness: this compound is unique due to the presence of the tert-butyl group, which provides steric hindrance and influences the reactivity and properties of the compound. This makes it particularly useful in the synthesis of peptides with specific structural and functional properties .
Properties
IUPAC Name |
(2S)-2-(9H-fluoren-9-ylmethoxycarbonylamino)-3,3-dimethylbutanoic acid | |
---|---|---|
Source | PubChem | |
URL | https://pubchem.ncbi.nlm.nih.gov | |
Description | Data deposited in or computed by PubChem | |
InChI |
InChI=1S/C21H23NO4/c1-21(2,3)18(19(23)24)22-20(25)26-12-17-15-10-6-4-8-13(15)14-9-5-7-11-16(14)17/h4-11,17-18H,12H2,1-3H3,(H,22,25)(H,23,24)/t18-/m1/s1 | |
Source | PubChem | |
URL | https://pubchem.ncbi.nlm.nih.gov | |
Description | Data deposited in or computed by PubChem | |
InChI Key |
VZOHGJIGTNUNNC-GOSISDBHSA-N | |
Source | PubChem | |
URL | https://pubchem.ncbi.nlm.nih.gov | |
Description | Data deposited in or computed by PubChem | |
Canonical SMILES |
CC(C)(C)C(C(=O)O)NC(=O)OCC1C2=CC=CC=C2C3=CC=CC=C13 | |
Source | PubChem | |
URL | https://pubchem.ncbi.nlm.nih.gov | |
Description | Data deposited in or computed by PubChem | |
Isomeric SMILES |
CC(C)(C)[C@@H](C(=O)O)NC(=O)OCC1C2=CC=CC=C2C3=CC=CC=C13 | |
Source | PubChem | |
URL | https://pubchem.ncbi.nlm.nih.gov | |
Description | Data deposited in or computed by PubChem | |
Molecular Formula |
C21H23NO4 | |
Source | PubChem | |
URL | https://pubchem.ncbi.nlm.nih.gov | |
Description | Data deposited in or computed by PubChem | |
DSSTOX Substance ID |
DTXSID70359658 | |
Record name | Fmoc-L-tert-leucine | |
Source | EPA DSSTox | |
URL | https://comptox.epa.gov/dashboard/DTXSID70359658 | |
Description | DSSTox provides a high quality public chemistry resource for supporting improved predictive toxicology. | |
Molecular Weight |
353.4 g/mol | |
Source | PubChem | |
URL | https://pubchem.ncbi.nlm.nih.gov | |
Description | Data deposited in or computed by PubChem | |
CAS No. |
132684-60-7 | |
Record name | Fmoc-L-tert-leucine | |
Source | EPA DSSTox | |
URL | https://comptox.epa.gov/dashboard/DTXSID70359658 | |
Description | DSSTox provides a high quality public chemistry resource for supporting improved predictive toxicology. | |
Record name | (2S)-2-(9H-fluoren-9-ylmethoxycarbonylamino)-3,3-dimethylbutanoic acid | |
Source | European Chemicals Agency (ECHA) | |
URL | https://echa.europa.eu/information-on-chemicals | |
Description | The European Chemicals Agency (ECHA) is an agency of the European Union which is the driving force among regulatory authorities in implementing the EU's groundbreaking chemicals legislation for the benefit of human health and the environment as well as for innovation and competitiveness. | |
Explanation | Use of the information, documents and data from the ECHA website is subject to the terms and conditions of this Legal Notice, and subject to other binding limitations provided for under applicable law, the information, documents and data made available on the ECHA website may be reproduced, distributed and/or used, totally or in part, for non-commercial purposes provided that ECHA is acknowledged as the source: "Source: European Chemicals Agency, http://echa.europa.eu/". Such acknowledgement must be included in each copy of the material. ECHA permits and encourages organisations and individuals to create links to the ECHA website under the following cumulative conditions: Links can only be made to webpages that provide a link to the Legal Notice page. | |
Retrosynthesis Analysis
AI-Powered Synthesis Planning: Our tool employs the Template_relevance Pistachio, Template_relevance Bkms_metabolic, Template_relevance Pistachio_ringbreaker, Template_relevance Reaxys, Template_relevance Reaxys_biocatalysis model, leveraging a vast database of chemical reactions to predict feasible synthetic routes.
One-Step Synthesis Focus: Specifically designed for one-step synthesis, it provides concise and direct routes for your target compounds, streamlining the synthesis process.
Accurate Predictions: Utilizing the extensive PISTACHIO, BKMS_METABOLIC, PISTACHIO_RINGBREAKER, REAXYS, REAXYS_BIOCATALYSIS database, our tool offers high-accuracy predictions, reflecting the latest in chemical research and data.
Strategy Settings
Precursor scoring | Relevance Heuristic |
---|---|
Min. plausibility | 0.01 |
Model | Template_relevance |
Template Set | Pistachio/Bkms_metabolic/Pistachio_ringbreaker/Reaxys/Reaxys_biocatalysis |
Top-N result to add to graph | 6 |
Feasible Synthetic Routes
Disclaimer and Information on In-Vitro Research Products
Please be aware that all articles and product information presented on BenchChem are intended solely for informational purposes. The products available for purchase on BenchChem are specifically designed for in-vitro studies, which are conducted outside of living organisms. In-vitro studies, derived from the Latin term "in glass," involve experiments performed in controlled laboratory settings using cells or tissues. It is important to note that these products are not categorized as medicines or drugs, and they have not received approval from the FDA for the prevention, treatment, or cure of any medical condition, ailment, or disease. We must emphasize that any form of bodily introduction of these products into humans or animals is strictly prohibited by law. It is essential to adhere to these guidelines to ensure compliance with legal and ethical standards in research and experimentation.