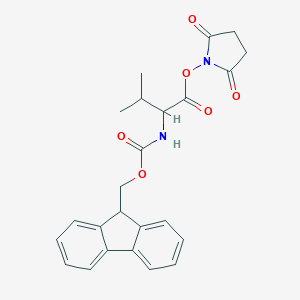
Fmoc-val-osu
Overview
Description
N-alpha-(9-Fluorenylmethyloxycarbonyl)-L-valine succinimidyl ester: , commonly known as Fmoc-val-osu, is a derivative of valine. It is widely used in peptide synthesis, particularly in solid-phase peptide synthesis techniques. The compound is characterized by the presence of the fluorenylmethyloxycarbonyl (Fmoc) protecting group, which is base-labile and used to protect the amine group of amino acids during synthesis .
Mechanism of Action
Target of Action
The primary target of Fmoc-val-osu is the amine group of amino acids . The compound is used as a protecting group for amines in organic synthesis .
Mode of Action
This compound operates by protecting the amine group of amino acids during peptide synthesis . The Fmoc group can be introduced by reacting the amine with fluorenylmethyloxycarbonyl chloride (Fmoc-Cl), or through 9-fluorenylmethylsuccinimidyl carbonate (Fmoc-OSu), which may itself be obtained by the reaction of Fmoc-Cl with the dicyclohexylammonium salt of N-hydroxysuccinimide .
Biochemical Pathways
The this compound compound plays a crucial role in the solid-phase peptide synthesis (SPPS) pathway .
Pharmacokinetics
The Fmoc group is rapidly removed by base . Piperidine is usually preferred for Fmoc group removal as it forms a stable adduct with the dibenzofulvene byproduct, preventing it from reacting with the substrate . A typical SPPS Fmoc deprotection is performed with a solution of 20% piperidine in N,N-dimethylformamide (DMF) .
Result of Action
The result of the action of this compound is the formation of peptides . The compound allows for the very rapid and highly efficient synthesis of peptides, including ones of significant size and complexity .
Action Environment
The action of this compound is influenced by the chemical environment . For instance, the Fmoc group has an approximate half-life of 6 seconds in a solution of 20% piperidine in DMF . Additionally, the use of strictly controlled piperidine can be avoided by using a cocktail of 5% piperazine, 1% DBU, and 1% formic acid in DMF .
Biochemical Analysis
Biochemical Properties
Fmoc-val-osu plays a significant role in biochemical reactions, particularly in the field of peptide synthesis . The Fmoc group serves as a protecting group for amines, which can be introduced by reacting the amine with fluorenylmethyloxycarbonyl chloride (Fmoc-Cl) or through 9-fluorenylmethylsuccinimidyl carbonate (Fmoc-OSu) . This compound interacts with various enzymes, proteins, and other biomolecules during these processes .
Cellular Effects
The cellular effects of this compound are primarily observed in the context of peptide synthesis and proteomics studies . It influences cell function by contributing to the formation of peptides, which can impact cell signaling pathways, gene expression, and cellular metabolism .
Molecular Mechanism
The molecular mechanism of this compound involves its role as a protecting group for amines in peptide synthesis . It exerts its effects at the molecular level through binding interactions with biomolecules, and changes in gene expression during peptide synthesis .
Temporal Effects in Laboratory Settings
In laboratory settings, the effects of this compound can change over time. For instance, the Fmoc group is rapidly removed by base, with piperidine usually preferred for Fmoc group removal . This process influences the stability and degradation of the compound, and can have long-term effects on cellular function in in vitro or in vivo studies .
Metabolic Pathways
This compound is involved in the metabolic pathways related to peptide synthesis . It interacts with enzymes and cofactors during these processes, and can influence metabolic flux or metabolite levels. Detailed information on the specific metabolic pathways involving this compound is currently limited.
Preparation Methods
Synthetic Routes and Reaction Conditions:
Fmoc-Val-OSu Synthesis: The synthesis of this compound typically involves the reaction of N-alpha-(9-fluorenylmethyloxycarbonyl)-L-valine with N-hydroxysuccinimide in the presence of a coupling agent such as dicyclohexylcarbodiimide (DCC).
Industrial Production Methods: Industrial production of this compound follows similar synthetic routes but on a larger scale.
Chemical Reactions Analysis
**Types of Reactions:
Properties
IUPAC Name |
(2,5-dioxopyrrolidin-1-yl) 2-(9H-fluoren-9-ylmethoxycarbonylamino)-3-methylbutanoate | |
---|---|---|
Details | Computed by Lexichem TK 2.7.0 (PubChem release 2021.05.07) | |
Source | PubChem | |
URL | https://pubchem.ncbi.nlm.nih.gov | |
Description | Data deposited in or computed by PubChem | |
InChI |
InChI=1S/C24H24N2O6/c1-14(2)22(23(29)32-26-20(27)11-12-21(26)28)25-24(30)31-13-19-17-9-5-3-7-15(17)16-8-4-6-10-18(16)19/h3-10,14,19,22H,11-13H2,1-2H3,(H,25,30) | |
Details | Computed by InChI 1.0.6 (PubChem release 2021.05.07) | |
Source | PubChem | |
URL | https://pubchem.ncbi.nlm.nih.gov | |
Description | Data deposited in or computed by PubChem | |
InChI Key |
JPJMNCROLRPFHI-UHFFFAOYSA-N | |
Details | Computed by InChI 1.0.6 (PubChem release 2021.05.07) | |
Source | PubChem | |
URL | https://pubchem.ncbi.nlm.nih.gov | |
Description | Data deposited in or computed by PubChem | |
Canonical SMILES |
CC(C)C(C(=O)ON1C(=O)CCC1=O)NC(=O)OCC2C3=CC=CC=C3C4=CC=CC=C24 | |
Details | Computed by OEChem 2.3.0 (PubChem release 2021.05.07) | |
Source | PubChem | |
URL | https://pubchem.ncbi.nlm.nih.gov | |
Description | Data deposited in or computed by PubChem | |
Molecular Formula |
C24H24N2O6 | |
Details | Computed by PubChem 2.1 (PubChem release 2021.05.07) | |
Source | PubChem | |
URL | https://pubchem.ncbi.nlm.nih.gov | |
Description | Data deposited in or computed by PubChem | |
DSSTOX Substance ID |
DTXSID50609224 | |
Record name | 2,5-Dioxopyrrolidin-1-yl N-{[(9H-fluoren-9-yl)methoxy]carbonyl}valinate | |
Source | EPA DSSTox | |
URL | https://comptox.epa.gov/dashboard/DTXSID50609224 | |
Description | DSSTox provides a high quality public chemistry resource for supporting improved predictive toxicology. | |
Molecular Weight |
436.5 g/mol | |
Details | Computed by PubChem 2.1 (PubChem release 2021.05.07) | |
Source | PubChem | |
URL | https://pubchem.ncbi.nlm.nih.gov | |
Description | Data deposited in or computed by PubChem | |
CAS No. |
130878-68-1 | |
Record name | 2,5-Dioxopyrrolidin-1-yl N-{[(9H-fluoren-9-yl)methoxy]carbonyl}valinate | |
Source | EPA DSSTox | |
URL | https://comptox.epa.gov/dashboard/DTXSID50609224 | |
Description | DSSTox provides a high quality public chemistry resource for supporting improved predictive toxicology. | |
Retrosynthesis Analysis
AI-Powered Synthesis Planning: Our tool employs the Template_relevance Pistachio, Template_relevance Bkms_metabolic, Template_relevance Pistachio_ringbreaker, Template_relevance Reaxys, Template_relevance Reaxys_biocatalysis model, leveraging a vast database of chemical reactions to predict feasible synthetic routes.
One-Step Synthesis Focus: Specifically designed for one-step synthesis, it provides concise and direct routes for your target compounds, streamlining the synthesis process.
Accurate Predictions: Utilizing the extensive PISTACHIO, BKMS_METABOLIC, PISTACHIO_RINGBREAKER, REAXYS, REAXYS_BIOCATALYSIS database, our tool offers high-accuracy predictions, reflecting the latest in chemical research and data.
Strategy Settings
Precursor scoring | Relevance Heuristic |
---|---|
Min. plausibility | 0.01 |
Model | Template_relevance |
Template Set | Pistachio/Bkms_metabolic/Pistachio_ringbreaker/Reaxys/Reaxys_biocatalysis |
Top-N result to add to graph | 6 |
Feasible Synthetic Routes
Disclaimer and Information on In-Vitro Research Products
Please be aware that all articles and product information presented on BenchChem are intended solely for informational purposes. The products available for purchase on BenchChem are specifically designed for in-vitro studies, which are conducted outside of living organisms. In-vitro studies, derived from the Latin term "in glass," involve experiments performed in controlled laboratory settings using cells or tissues. It is important to note that these products are not categorized as medicines or drugs, and they have not received approval from the FDA for the prevention, treatment, or cure of any medical condition, ailment, or disease. We must emphasize that any form of bodily introduction of these products into humans or animals is strictly prohibited by law. It is essential to adhere to these guidelines to ensure compliance with legal and ethical standards in research and experimentation.