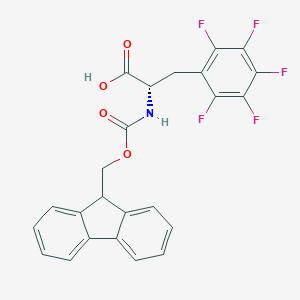
Fmoc-pentafluoro-L-phenylalanine
Overview
Description
Synthesis Analysis
Fmoc-pentafluoro-L-phenylalanine is used in Fmoc solid-phase peptide synthesis . The key role of Fmoc, Fmoc and phenylalanine covalent linkage, flexibility of phe side chain, pH, and buffer ions in self-assembly of FmocF to gel formation is described .Molecular Structure Analysis
The molecular formula of this compound is C24H16F5NO4 . Its molecular weight is 477.4 g/mol . The InChI string is InChI=1S/C24H16F5NO4/c25-18-15 (19 (26)21 (28)22 (29)20 (18)27)9-17 (23 (31)32)30-24 (33)34-10-16-13-7-3-1-5-11 (13)12-6-2-4-8-14 (12)16/h1-8,16-17H,9-10H2, (H,30,33) (H,31,32)/t17-/m0/s1 .Chemical Reactions Analysis
This compound is suitable for Fmoc solid-phase peptide synthesis . The self-organization and applications of Fmoc-modified simple biomolecules have not been extensively summarized .Physical And Chemical Properties Analysis
This compound has a molecular weight of 477.38 . Its optical activity is [α]/D -20.5±1.0°, c = 1 in methanol .Scientific Research Applications
Antibacterial Composite Materials : Fmoc-pentafluoro-L-phenylalanine nanoassemblies exhibit significant antibacterial capabilities and have been integrated into resin-based composites. These composites inhibit bacterial growth without affecting mammalian cell lines and do not compromise mechanical and optical properties, suggesting potential in biomedical materials (Schnaider et al., 2019).
Hydrogel Formation : this compound has been explored for its self-assembly and hydrogelation properties. Its efficient self-assembly into entangled fibrillar structures forms rigid supramolecular gels, important for novel small molecule hydrogelators (Ryan et al., 2010).
Hydrogelation Behavior : The influence of side-chain halogenation on the self-assembly and hydrogelation of Fmoc-phenylalanine derivatives, including this compound, was studied. Halogenation enhances self-assembly into amyloid-like fibrils, affecting the rate of self-assembly and the rheological properties of the resulting hydrogel (Ryan et al., 2010).
Mass Spectrometry Applications : this compound has been used in studies involving time-of-flight secondary ion mass spectrometry (ToF-SIMS), demonstrating strong and unusual matrix effects in organic SIMS, highlighting its utility in quantitative analysis and compositional variation assessment in materials (Shard et al., 2015).
Modification Effects on Self-assembly : Studies on the modification of the C-terminal of fluorinated Fmoc-Phe derivatives, including this compound, have provided insights into how monomer/solvent interactions influence self-assembly and hydrogelation. This research aids in the development of amino acid hydrogelators for complex media (Ryan et al., 2011).
Nanotube Formation in Hydrogels : Cation-modified Fmoc-phenylalanine derivatives, including this compound, can self-assemble into hydrogel networks and form nanotube structures, a property unique to these cationic derivatives. This research offers insights into the self-assembly pathways of Fmoc-Phe derivatives (Rajbhandary et al., 2017).
Peptide Synthesis : this compound has been utilized in the synthesis of peptides, like in the case of erythro-N-Boc-β-mercapto-l-phenylalanine, demonstrating its compatibility with reactive side chains in peptide ligation processes (Crich & Banerjee, 2007).
Mechanism of Action
- Fmoc-L-Pentafluorophenylalanine primarily targets biological systems, particularly cellular components involved in self-assembly and functional material fabrication .
- Fmoc-L-Pentafluorophenylalanine self-assembles through hydrophobic interactions, driven by the fluorenyl ring and steric optimization from the linker (methoxycarbonyl group) .
- The compound’s self-assembly affects various pathways related to cell culture, bio-templating, optical properties, drug delivery, catalysis, and therapeutic applications .
- Fmoc-L-Pentafluorophenylalanine’s pharmacokinetic properties include its hydrophobicity and aromaticity, which impact bioavailability .
Target of Action
Mode of Action
Biochemical Pathways
Pharmacokinetics
Result of Action
Action Environment
Safety and Hazards
Fmoc-pentafluoro-L-phenylalanine is classified as Aquatic Chronic 4 according to hazard classifications . It is recommended to avoid breathing mist, gas or vapours, avoid contacting with skin and eye, use personal protective equipment, wear chemical impermeable gloves, ensure adequate ventilation, remove all sources of ignition, evacuate personnel to safe areas, and keep people away from and upwind of spill/leak .
Future Directions
The rapid advancement of peptide- and amino-acid-based nanotechnology offers new approaches for the development of biomedical materials . The utilization of fluorenylmethyloxycarbonyl (Fmoc)-decorated self-assembling building blocks for antibacterial and anti-inflammatory purposes represents promising advancements in this field .
Biochemical Analysis
Biochemical Properties
Fmoc-pentafluoro-L-phenylalanine plays a significant role in biochemical reactions, particularly in peptide synthesis . It is involved in Fmoc solid-phase peptide synthesis, a method used to chemically synthesize peptides . This compound interacts with various enzymes, proteins, and other biomolecules in these reactions . The nature of these interactions is largely governed by the aromaticity of the Fmoc group, which promotes the association of peptide building blocks .
Cellular Effects
The effects of this compound on cells and cellular processes are diverse. For instance, Fmoc-modified amino acids, including this compound, have been found to have antimicrobial properties specific to Gram-positive bacteria . The compound influences cell function by interacting with cellular components and affecting various cellular processes .
Molecular Mechanism
The mechanism of action of this compound at the molecular level involves several binding interactions with biomolecules. The compound exerts its effects through these interactions, which can lead to changes in gene expression . The Fmoc group in the compound plays a key role in these interactions, contributing to the self-assembly of the compound .
properties
IUPAC Name |
(2S)-2-(9H-fluoren-9-ylmethoxycarbonylamino)-3-(2,3,4,5,6-pentafluorophenyl)propanoic acid | |
---|---|---|
Source | PubChem | |
URL | https://pubchem.ncbi.nlm.nih.gov | |
Description | Data deposited in or computed by PubChem | |
InChI |
InChI=1S/C24H16F5NO4/c25-18-15(19(26)21(28)22(29)20(18)27)9-17(23(31)32)30-24(33)34-10-16-13-7-3-1-5-11(13)12-6-2-4-8-14(12)16/h1-8,16-17H,9-10H2,(H,30,33)(H,31,32)/t17-/m0/s1 | |
Source | PubChem | |
URL | https://pubchem.ncbi.nlm.nih.gov | |
Description | Data deposited in or computed by PubChem | |
InChI Key |
DLOGILOIJKBYKA-KRWDZBQOSA-N | |
Source | PubChem | |
URL | https://pubchem.ncbi.nlm.nih.gov | |
Description | Data deposited in or computed by PubChem | |
Canonical SMILES |
C1=CC=C2C(=C1)C(C3=CC=CC=C32)COC(=O)NC(CC4=C(C(=C(C(=C4F)F)F)F)F)C(=O)O | |
Source | PubChem | |
URL | https://pubchem.ncbi.nlm.nih.gov | |
Description | Data deposited in or computed by PubChem | |
Isomeric SMILES |
C1=CC=C2C(=C1)C(C3=CC=CC=C32)COC(=O)N[C@@H](CC4=C(C(=C(C(=C4F)F)F)F)F)C(=O)O | |
Source | PubChem | |
URL | https://pubchem.ncbi.nlm.nih.gov | |
Description | Data deposited in or computed by PubChem | |
Molecular Formula |
C24H16F5NO4 | |
Source | PubChem | |
URL | https://pubchem.ncbi.nlm.nih.gov | |
Description | Data deposited in or computed by PubChem | |
DSSTOX Substance ID |
DTXSID60942735 | |
Record name | N-{[(9H-Fluoren-9-yl)methoxy](hydroxy)methylidene}-2,3,4,5,6-pentafluorophenylalanine | |
Source | EPA DSSTox | |
URL | https://comptox.epa.gov/dashboard/DTXSID60942735 | |
Description | DSSTox provides a high quality public chemistry resource for supporting improved predictive toxicology. | |
Molecular Weight |
477.4 g/mol | |
Source | PubChem | |
URL | https://pubchem.ncbi.nlm.nih.gov | |
Description | Data deposited in or computed by PubChem | |
CAS RN |
205526-32-5 | |
Record name | N-{[(9H-Fluoren-9-yl)methoxy](hydroxy)methylidene}-2,3,4,5,6-pentafluorophenylalanine | |
Source | EPA DSSTox | |
URL | https://comptox.epa.gov/dashboard/DTXSID60942735 | |
Description | DSSTox provides a high quality public chemistry resource for supporting improved predictive toxicology. | |
Record name | Fmoc-pentafluoro-L-phenylalanine | |
Source | European Chemicals Agency (ECHA) | |
URL | https://echa.europa.eu/information-on-chemicals | |
Description | The European Chemicals Agency (ECHA) is an agency of the European Union which is the driving force among regulatory authorities in implementing the EU's groundbreaking chemicals legislation for the benefit of human health and the environment as well as for innovation and competitiveness. | |
Explanation | Use of the information, documents and data from the ECHA website is subject to the terms and conditions of this Legal Notice, and subject to other binding limitations provided for under applicable law, the information, documents and data made available on the ECHA website may be reproduced, distributed and/or used, totally or in part, for non-commercial purposes provided that ECHA is acknowledged as the source: "Source: European Chemicals Agency, http://echa.europa.eu/". Such acknowledgement must be included in each copy of the material. ECHA permits and encourages organisations and individuals to create links to the ECHA website under the following cumulative conditions: Links can only be made to webpages that provide a link to the Legal Notice page. | |
Retrosynthesis Analysis
AI-Powered Synthesis Planning: Our tool employs the Template_relevance Pistachio, Template_relevance Bkms_metabolic, Template_relevance Pistachio_ringbreaker, Template_relevance Reaxys, Template_relevance Reaxys_biocatalysis model, leveraging a vast database of chemical reactions to predict feasible synthetic routes.
One-Step Synthesis Focus: Specifically designed for one-step synthesis, it provides concise and direct routes for your target compounds, streamlining the synthesis process.
Accurate Predictions: Utilizing the extensive PISTACHIO, BKMS_METABOLIC, PISTACHIO_RINGBREAKER, REAXYS, REAXYS_BIOCATALYSIS database, our tool offers high-accuracy predictions, reflecting the latest in chemical research and data.
Strategy Settings
Precursor scoring | Relevance Heuristic |
---|---|
Min. plausibility | 0.01 |
Model | Template_relevance |
Template Set | Pistachio/Bkms_metabolic/Pistachio_ringbreaker/Reaxys/Reaxys_biocatalysis |
Top-N result to add to graph | 6 |
Feasible Synthetic Routes
Q & A
Q1: What is the significance of Fmoc-PFLPA in materials science research?
A1: Fmoc-PFLPA serves as a model compound in studies focusing on the characterization and analysis of organic materials, especially in thin films and layered structures. Its distinct chemical properties, different from compounds like Irganox 1010 and Irganox 1098, make it valuable for studying matrix effects in techniques like Time-of-Flight Secondary Ion Mass Spectrometry (ToF-SIMS) [, ].
Q2: Why are matrix effects important in analyzing Fmoc-PFLPA-containing materials?
A2: Matrix effects in ToF-SIMS can lead to inaccuracies when determining the composition of mixtures containing Fmoc-PFLPA [, , ]. These effects arise from the influence of neighboring molecules on the ionization and detection of secondary ions, making it challenging to directly correlate signal intensity with concentration. This is particularly relevant in depth profiling studies using argon cluster ions, where accurate quantification of Fmoc-PFLPA within a matrix is crucial [, ].
Q3: What strategies are used to address matrix effects in the analysis of Fmoc-PFLPA?
A3: Researchers employ various strategies to mitigate matrix effects. One approach involves using specific secondary ions less susceptible to these effects during depth profiling []. Another method utilizes correction algorithms, often based on measuring known standards or comparing positive and negative secondary ion data, to improve the accuracy of compositional analysis [, ]. Additionally, advanced data analysis techniques like autoencoders and artificial neural networks (ANN) are being explored to establish quantitative relationships between ion intensities and concentrations, effectively accounting for matrix effects [].
Q4: How is Fmoc-PFLPA used in developing reference materials for organic thin films?
A4: Fmoc-PFLPA, often in combination with Irganox 1010, is used to create well-defined layered structures on silicon substrates. These structures serve as reference materials for calibrating analytical techniques, particularly X-ray reflectometry, used to determine the thickness of organic nanolayers []. The precise control over layer composition and thickness in these reference materials allows for traceable measurements linked to the International System of Units (SI), enhancing the accuracy and reliability of thickness determination in organic thin films.
Q5: Beyond material science, are there other applications of Fmoc-PFLPA?
A5: While primarily known for its role in material analysis, Fmoc-PFLPA serves as a building block for creating self-assembling nanoassemblies [, ]. These nanoassemblies, driven by the Fmoc group's self-assembly properties, exhibit antibacterial capabilities and are being investigated for incorporation into composite materials, potentially enhancing their antibacterial properties without compromising mechanical integrity. This highlights the potential for Fmoc-PFLPA to contribute to the development of novel biomedical materials.
Disclaimer and Information on In-Vitro Research Products
Please be aware that all articles and product information presented on BenchChem are intended solely for informational purposes. The products available for purchase on BenchChem are specifically designed for in-vitro studies, which are conducted outside of living organisms. In-vitro studies, derived from the Latin term "in glass," involve experiments performed in controlled laboratory settings using cells or tissues. It is important to note that these products are not categorized as medicines or drugs, and they have not received approval from the FDA for the prevention, treatment, or cure of any medical condition, ailment, or disease. We must emphasize that any form of bodily introduction of these products into humans or animals is strictly prohibited by law. It is essential to adhere to these guidelines to ensure compliance with legal and ethical standards in research and experimentation.