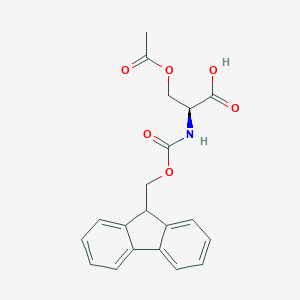
Fmoc-Ser(Ac)-OH
Overview
Description
Fmoc-Ser(Ac)-OH, also known as 9-fluorenylmethyloxycarbonyl-O-acetyl-L-serine, is a derivative of the amino acid serine. It is commonly used in peptide synthesis due to its ability to protect the amino group during the synthesis process. The Fmoc group is a popular protecting group in solid-phase peptide synthesis because it can be removed under mildly basic conditions, which helps to prevent unwanted side reactions.
Mechanism of Action
Target of Action
Fmoc-Ser(Ac)-OH, a derivative of the amino acid serine, is primarily used as a building block in peptide synthesis . The primary targets of this compound are the peptide chains being synthesized, where it contributes to the formation of specific peptide sequences .
Mode of Action
The mode of action of this compound involves its incorporation into peptide chains through a process known as Fmoc/tBu solid-phase synthesis . This synthetic strategy involves a solid polymeric protecting group and allows the use of an excess of reagents to achieve quantitative yields . The Fmoc group serves as a temporary protecting group for the amino group during peptide chain assembly .
Biochemical Pathways
The biochemical pathways involved in the action of this compound are primarily those related to peptide synthesis. The compound plays a key role in the formation of peptide bonds, contributing to the assembly of complex peptide structures .
Pharmacokinetics
Its solubility and stability under various conditions are crucial for its effective use in peptide synthesis .
Result of Action
The result of this compound’s action is the successful synthesis of the desired peptide chains. These peptides can then be used in various applications, from research to the production of therapeutic agents .
Action Environment
The action of this compound is influenced by various environmental factors. For instance, pH and buffer ions play a role in the self-assembly of this compound to gel formation . Additionally, physical and thermal stimuli can be used for solubilizing this compound above the critical concentration to induce gel formation .
Biochemical Analysis
Biochemical Properties
Fmoc-Ser(Ac)-OH is known for its eminent self-assembly features, which are largely attributed to the inherent hydrophobicity and aromaticity of the Fmoc moiety . This property allows this compound to interact with various enzymes, proteins, and other biomolecules, promoting the association of building blocks .
Cellular Effects
The effects of this compound on cells and cellular processes are diverse. It influences cell function, including impacts on cell signaling pathways, gene expression, and cellular metabolism . The inherent hydrophobicity and aromaticity of the Fmoc moiety in this compound can promote the association of building blocks, which may influence various cellular processes .
Molecular Mechanism
The molecular mechanism of this compound involves its interaction with biomolecules at the molecular level. The Fmoc group in this compound can promote the association of building blocks, potentially influencing enzyme activity, biomolecular binding interactions, and changes in gene expression .
Temporal Effects in Laboratory Settings
In laboratory settings, the effects of this compound can change over time. Factors such as the product’s stability, degradation, and long-term effects on cellular function are important considerations in in vitro or in vivo studies .
Preparation Methods
Synthetic Routes and Reaction Conditions: The synthesis of Fmoc-Ser(Ac)-OH typically involves the protection of the serine hydroxyl group with an acetyl group, followed by the protection of the amino group with the Fmoc group. The general steps are as follows:
Acetylation of Serine: Serine is reacted with acetic anhydride in the presence of a base such as pyridine to form O-acetyl-L-serine.
Fmoc Protection: The O-acetyl-L-serine is then reacted with 9-fluorenylmethyloxycarbonyl chloride (Fmoc-Cl) in the presence of a base such as sodium bicarbonate to form this compound.
Industrial Production Methods: In industrial settings, the production of this compound follows similar synthetic routes but on a larger scale. The process involves the use of automated peptide synthesizers that can handle large quantities of reagents and solvents, ensuring high purity and yield of the final product.
Chemical Reactions Analysis
Types of Reactions: Fmoc-Ser(Ac)-OH undergoes several types of chemical reactions, including:
Deprotection Reactions: The Fmoc group can be removed using a base such as piperidine, revealing the free amino group.
Coupling Reactions: The free amino group can then participate in peptide bond formation with other amino acids or peptides.
Hydrolysis: The acetyl group can be hydrolyzed under acidic or basic conditions to yield free serine.
Common Reagents and Conditions:
Deprotection: Piperidine in dimethylformamide (DMF) is commonly used for Fmoc deprotection.
Coupling: Reagents such as N,N’-diisopropylcarbodiimide (DIC) and hydroxybenzotriazole (HOBt) are used for peptide coupling reactions.
Hydrolysis: Acidic conditions (e.g., hydrochloric acid) or basic conditions (e.g., sodium hydroxide) can be used for acetyl group hydrolysis.
Major Products:
Deprotection: Removal of the Fmoc group yields O-acetyl-L-serine.
Coupling: Formation of peptide bonds with other amino acids or peptides.
Hydrolysis: Free serine is obtained after acetyl group removal.
Scientific Research Applications
Fmoc-Ser(Ac)-OH has a wide range of applications in scientific research, including:
Peptide Synthesis: It is used as a building block in the synthesis of peptides and proteins.
Drug Development: Peptides synthesized using this compound can be used in the development of therapeutic agents.
Biomaterials: this compound is used in the design of biomaterials for tissue engineering and regenerative medicine.
Biological Studies: It is used in the study of protein-protein interactions and enzyme mechanisms.
Comparison with Similar Compounds
Fmoc-Ser(tBu)-OH: This compound has a tert-butyl group protecting the hydroxyl group instead of an acetyl group.
Fmoc-Thr(Ac)-OH: Similar to Fmoc-Ser(Ac)-OH, but with threonine instead of serine.
Fmoc-Tyr(tBu)-OH: This compound has a tert-butyl group protecting the hydroxyl group of tyrosine.
Uniqueness: this compound is unique due to its specific protecting groups, which provide distinct reactivity and stability during peptide synthesis. The acetyl group offers a different level of protection and deprotection conditions compared to other protecting groups like tert-butyl.
Properties
IUPAC Name |
(2S)-3-acetyloxy-2-(9H-fluoren-9-ylmethoxycarbonylamino)propanoic acid | |
---|---|---|
Source | PubChem | |
URL | https://pubchem.ncbi.nlm.nih.gov | |
Description | Data deposited in or computed by PubChem | |
InChI |
InChI=1S/C20H19NO6/c1-12(22)26-11-18(19(23)24)21-20(25)27-10-17-15-8-4-2-6-13(15)14-7-3-5-9-16(14)17/h2-9,17-18H,10-11H2,1H3,(H,21,25)(H,23,24)/t18-/m0/s1 | |
Source | PubChem | |
URL | https://pubchem.ncbi.nlm.nih.gov | |
Description | Data deposited in or computed by PubChem | |
InChI Key |
HSGIKRPBGCJRDB-SFHVURJKSA-N | |
Source | PubChem | |
URL | https://pubchem.ncbi.nlm.nih.gov | |
Description | Data deposited in or computed by PubChem | |
Canonical SMILES |
CC(=O)OCC(C(=O)O)NC(=O)OCC1C2=CC=CC=C2C3=CC=CC=C13 | |
Source | PubChem | |
URL | https://pubchem.ncbi.nlm.nih.gov | |
Description | Data deposited in or computed by PubChem | |
Isomeric SMILES |
CC(=O)OC[C@@H](C(=O)O)NC(=O)OCC1C2=CC=CC=C2C3=CC=CC=C13 | |
Source | PubChem | |
URL | https://pubchem.ncbi.nlm.nih.gov | |
Description | Data deposited in or computed by PubChem | |
Molecular Formula |
C20H19NO6 | |
Source | PubChem | |
URL | https://pubchem.ncbi.nlm.nih.gov | |
Description | Data deposited in or computed by PubChem | |
DSSTOX Substance ID |
DTXSID20427038 | |
Record name | Fmoc-L-Ser(Ac) | |
Source | EPA DSSTox | |
URL | https://comptox.epa.gov/dashboard/DTXSID20427038 | |
Description | DSSTox provides a high quality public chemistry resource for supporting improved predictive toxicology. | |
Molecular Weight |
369.4 g/mol | |
Source | PubChem | |
URL | https://pubchem.ncbi.nlm.nih.gov | |
Description | Data deposited in or computed by PubChem | |
CAS No. |
171778-17-9 | |
Record name | O-Acetyl-N-[(9H-fluoren-9-ylmethoxy)carbonyl]-L-serine | |
Source | CAS Common Chemistry | |
URL | https://commonchemistry.cas.org/detail?cas_rn=171778-17-9 | |
Description | CAS Common Chemistry is an open community resource for accessing chemical information. Nearly 500,000 chemical substances from CAS REGISTRY cover areas of community interest, including common and frequently regulated chemicals, and those relevant to high school and undergraduate chemistry classes. This chemical information, curated by our expert scientists, is provided in alignment with our mission as a division of the American Chemical Society. | |
Explanation | The data from CAS Common Chemistry is provided under a CC-BY-NC 4.0 license, unless otherwise stated. | |
Record name | NSC 778228 | |
Source | ChemIDplus | |
URL | https://pubchem.ncbi.nlm.nih.gov/substance/?source=chemidplus&sourceid=0171778179 | |
Description | ChemIDplus is a free, web search system that provides access to the structure and nomenclature authority files used for the identification of chemical substances cited in National Library of Medicine (NLM) databases, including the TOXNET system. | |
Record name | Fmoc-L-Ser(Ac) | |
Source | EPA DSSTox | |
URL | https://comptox.epa.gov/dashboard/DTXSID20427038 | |
Description | DSSTox provides a high quality public chemistry resource for supporting improved predictive toxicology. | |
Retrosynthesis Analysis
AI-Powered Synthesis Planning: Our tool employs the Template_relevance Pistachio, Template_relevance Bkms_metabolic, Template_relevance Pistachio_ringbreaker, Template_relevance Reaxys, Template_relevance Reaxys_biocatalysis model, leveraging a vast database of chemical reactions to predict feasible synthetic routes.
One-Step Synthesis Focus: Specifically designed for one-step synthesis, it provides concise and direct routes for your target compounds, streamlining the synthesis process.
Accurate Predictions: Utilizing the extensive PISTACHIO, BKMS_METABOLIC, PISTACHIO_RINGBREAKER, REAXYS, REAXYS_BIOCATALYSIS database, our tool offers high-accuracy predictions, reflecting the latest in chemical research and data.
Strategy Settings
Precursor scoring | Relevance Heuristic |
---|---|
Min. plausibility | 0.01 |
Model | Template_relevance |
Template Set | Pistachio/Bkms_metabolic/Pistachio_ringbreaker/Reaxys/Reaxys_biocatalysis |
Top-N result to add to graph | 6 |
Feasible Synthetic Routes
Disclaimer and Information on In-Vitro Research Products
Please be aware that all articles and product information presented on BenchChem are intended solely for informational purposes. The products available for purchase on BenchChem are specifically designed for in-vitro studies, which are conducted outside of living organisms. In-vitro studies, derived from the Latin term "in glass," involve experiments performed in controlled laboratory settings using cells or tissues. It is important to note that these products are not categorized as medicines or drugs, and they have not received approval from the FDA for the prevention, treatment, or cure of any medical condition, ailment, or disease. We must emphasize that any form of bodily introduction of these products into humans or animals is strictly prohibited by law. It is essential to adhere to these guidelines to ensure compliance with legal and ethical standards in research and experimentation.