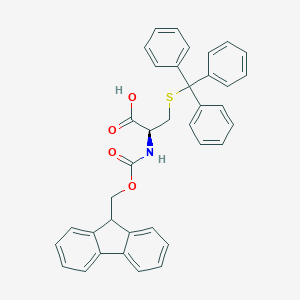
Fmoc-D-Cys(Trt)-OH
Overview
Description
Synthesis Analysis
Fmoc-D-Cys(Trt)-OH is used in peptide synthesis . The synthesis of glycoproteins is one of the grand challenges of organic chemistry and continues to stretch the frontiers of organic synthesis .Molecular Structure Analysis
The molecular formula of Fmoc-D-Cys(Trt)-OH is C37H31NO4S . Its molecular weight is 585.7 .Chemical Reactions Analysis
Fmoc-D-Cys(Trt)-OH is used in peptide synthesis . It has been used in the synthesis of mono- and bi-functionalized platinum (IV) complexes to target angiogenic tumor vasculature .Physical And Chemical Properties Analysis
Fmoc-D-Cys(Trt)-OH is a white powder or crystal . It is used in Fmoc solid-phase peptide synthesis .Scientific Research Applications
Fmoc-Cys(Trt)-OH is essential for the Fmoc/tBu strategy in peptide synthesis, providing compatibility with other Cys-protecting groups and aiding in the regioselective construction of disulfide bonds (Góngora-Benítez et al., 2012).
It exhibits excellent synthesis characteristics in Fmoc solid phase peptide synthesis, proving efficient in the synthesis of peptides like Somatostatin (Mccurdy, 1989).
The compound is prone to racemization during activation/coupling in Fmoc solid phase peptide synthesis, which can be mitigated by using a base-free activation step (Kaiser et al., 1996).
Fmoc-Cys(Trt)-OH plays a role in the synthesis of cyclic peptides, which have shown potential as anti-malarial agents (Fagúndez et al., 2018).
The compound is also useful in chemical protein synthesis, particularly when used as an N-masked group for cysteine in peptide thioester segments, facilitating the synthesis of proteins through native chemical ligations (Kar et al., 2020).
In the field of materials science, it is used in the development of new antibacterial composite materials, showcasing the integration of Fmoc-decorated self-assembling building blocks in biomedical applications (Schnaider et al., 2019).
Safety and Hazards
Mechanism of Action
Target of Action
Fmoc-D-Cys(Trt)-OH is primarily used in the field of peptide synthesis . Its primary targets are the amino acid sequences of peptides, where it serves as a building block in the formation of these sequences .
Mode of Action
Fmoc-D-Cys(Trt)-OH interacts with its targets through a process known as Fmoc-based solid phase synthesis . This process involves the formation of peptide bonds to create a chain of amino acids, resulting in the synthesis of peptide sequences . The Fmoc group serves as a protective group for the amino acid during synthesis, preventing unwanted side reactions .
Biochemical Pathways
The key biochemical pathway involved in the action of Fmoc-D-Cys(Trt)-OH is the native chemical ligation (NCL) pathway . This pathway allows for the convergent synthesis of proteins, revolutionizing the field of peptide synthesis . The compound plays a crucial role in this pathway as a thioester surrogate, enabling access to peptide thioesters .
Pharmacokinetics
For instance, the compound is stable under the repeated piperidine treatments used for Fmoc deprotection .
Result of Action
The result of Fmoc-D-Cys(Trt)-OH’s action is the successful synthesis of peptide sequences . These sequences can be used in the study of protein structure and function, the development of new pharmaceuticals, and various other applications in the field of biochemistry .
Action Environment
The action of Fmoc-D-Cys(Trt)-OH can be influenced by various environmental factors. For instance, the pH of the environment can affect the kinetics of the native chemical ligation reactions . Additionally, the compound’s propensity for racemization can be a concern under certain conditions . Therefore, careful control of the environment is necessary to ensure the compound’s effective action and stability .
properties
IUPAC Name |
(2S)-2-(9H-fluoren-9-ylmethoxycarbonylamino)-3-tritylsulfanylpropanoic acid | |
---|---|---|
Source | PubChem | |
URL | https://pubchem.ncbi.nlm.nih.gov | |
Description | Data deposited in or computed by PubChem | |
InChI |
InChI=1S/C37H31NO4S/c39-35(40)34(38-36(41)42-24-33-31-22-12-10-20-29(31)30-21-11-13-23-32(30)33)25-43-37(26-14-4-1-5-15-26,27-16-6-2-7-17-27)28-18-8-3-9-19-28/h1-23,33-34H,24-25H2,(H,38,41)(H,39,40)/t34-/m1/s1 | |
Source | PubChem | |
URL | https://pubchem.ncbi.nlm.nih.gov | |
Description | Data deposited in or computed by PubChem | |
InChI Key |
KLBPUVPNPAJWHZ-UUWRZZSWSA-N | |
Source | PubChem | |
URL | https://pubchem.ncbi.nlm.nih.gov | |
Description | Data deposited in or computed by PubChem | |
Canonical SMILES |
C1=CC=C(C=C1)C(C2=CC=CC=C2)(C3=CC=CC=C3)SCC(C(=O)O)NC(=O)OCC4C5=CC=CC=C5C6=CC=CC=C46 | |
Source | PubChem | |
URL | https://pubchem.ncbi.nlm.nih.gov | |
Description | Data deposited in or computed by PubChem | |
Isomeric SMILES |
C1=CC=C(C=C1)C(C2=CC=CC=C2)(C3=CC=CC=C3)SC[C@H](C(=O)O)NC(=O)OCC4C5=CC=CC=C5C6=CC=CC=C46 | |
Source | PubChem | |
URL | https://pubchem.ncbi.nlm.nih.gov | |
Description | Data deposited in or computed by PubChem | |
Molecular Formula |
C37H31NO4S | |
Source | PubChem | |
URL | https://pubchem.ncbi.nlm.nih.gov | |
Description | Data deposited in or computed by PubChem | |
DSSTOX Substance ID |
DTXSID90428234 | |
Record name | Fmoc-D-Cys(Trt)-OH | |
Source | EPA DSSTox | |
URL | https://comptox.epa.gov/dashboard/DTXSID90428234 | |
Description | DSSTox provides a high quality public chemistry resource for supporting improved predictive toxicology. | |
Molecular Weight |
585.7 g/mol | |
Source | PubChem | |
URL | https://pubchem.ncbi.nlm.nih.gov | |
Description | Data deposited in or computed by PubChem | |
Product Name |
Fmoc-D-Cys(Trt)-OH | |
CAS RN |
167015-11-4 | |
Record name | Fmoc-D-Cys(Trt)-OH | |
Source | EPA DSSTox | |
URL | https://comptox.epa.gov/dashboard/DTXSID90428234 | |
Description | DSSTox provides a high quality public chemistry resource for supporting improved predictive toxicology. | |
Record name | Fmoc-D-Cys(Trt)-OH | |
Source | European Chemicals Agency (ECHA) | |
URL | https://echa.europa.eu/information-on-chemicals | |
Description | The European Chemicals Agency (ECHA) is an agency of the European Union which is the driving force among regulatory authorities in implementing the EU's groundbreaking chemicals legislation for the benefit of human health and the environment as well as for innovation and competitiveness. | |
Explanation | Use of the information, documents and data from the ECHA website is subject to the terms and conditions of this Legal Notice, and subject to other binding limitations provided for under applicable law, the information, documents and data made available on the ECHA website may be reproduced, distributed and/or used, totally or in part, for non-commercial purposes provided that ECHA is acknowledged as the source: "Source: European Chemicals Agency, http://echa.europa.eu/". Such acknowledgement must be included in each copy of the material. ECHA permits and encourages organisations and individuals to create links to the ECHA website under the following cumulative conditions: Links can only be made to webpages that provide a link to the Legal Notice page. | |
Retrosynthesis Analysis
AI-Powered Synthesis Planning: Our tool employs the Template_relevance Pistachio, Template_relevance Bkms_metabolic, Template_relevance Pistachio_ringbreaker, Template_relevance Reaxys, Template_relevance Reaxys_biocatalysis model, leveraging a vast database of chemical reactions to predict feasible synthetic routes.
One-Step Synthesis Focus: Specifically designed for one-step synthesis, it provides concise and direct routes for your target compounds, streamlining the synthesis process.
Accurate Predictions: Utilizing the extensive PISTACHIO, BKMS_METABOLIC, PISTACHIO_RINGBREAKER, REAXYS, REAXYS_BIOCATALYSIS database, our tool offers high-accuracy predictions, reflecting the latest in chemical research and data.
Strategy Settings
Precursor scoring | Relevance Heuristic |
---|---|
Min. plausibility | 0.01 |
Model | Template_relevance |
Template Set | Pistachio/Bkms_metabolic/Pistachio_ringbreaker/Reaxys/Reaxys_biocatalysis |
Top-N result to add to graph | 6 |
Feasible Synthetic Routes
Disclaimer and Information on In-Vitro Research Products
Please be aware that all articles and product information presented on BenchChem are intended solely for informational purposes. The products available for purchase on BenchChem are specifically designed for in-vitro studies, which are conducted outside of living organisms. In-vitro studies, derived from the Latin term "in glass," involve experiments performed in controlled laboratory settings using cells or tissues. It is important to note that these products are not categorized as medicines or drugs, and they have not received approval from the FDA for the prevention, treatment, or cure of any medical condition, ailment, or disease. We must emphasize that any form of bodily introduction of these products into humans or animals is strictly prohibited by law. It is essential to adhere to these guidelines to ensure compliance with legal and ethical standards in research and experimentation.