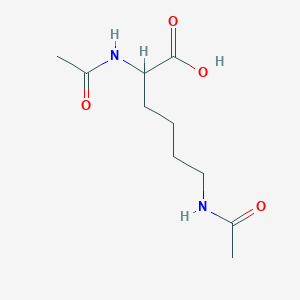
2,6-Diacetamidohexanoic acid
Overview
Description
Scientific Research Applications
Hexamethylene bisacetamide, a compound that induces differentiation in cancer cell lines, is metabolized into several compounds including 6-acetamidohexanoic acid. This metabolite and others are identified using gas chromatography-mass spectrometric analysis, highlighting the importance of these metabolites in cancer research (Callery et al., 1986).
6-Aminohexanoic acid, structurally related to 2,6-Diacetamidohexanoic acid, is used in chemical synthesis of modified peptides and in the polyamide synthetic fibers industry. It also functions as a linker in biologically active structures, demonstrating its versatility in chemical and biochemical applications (Markowska et al., 2021).
4-Aminohex-5-ynoic acid, an analogue of 4-aminobutyric acid and structurally similar to 2,6-Diacetamidohexanoic acid, shows potential as an inhibitor for bacterial glutamic acid decarboxylase, illustrating its importance in the development of antimicrobial agents (Jung et al., 1978).
The design and synthesis of 2-Aminobicyclo[3.1.0]hexane-2,6-dicarboxylic acid as a glutamate receptor agonist demonstrates the potential of compounds structurally related to 2,6-Diacetamidohexanoic acid in developing neurological disorder treatments (Monn et al., 1997).
Studies on alkylamides, including 6-acetamidohexanoic acid, have explored their ability to induce cell differentiation in leukemia cells, contributing to potential cancer therapies (Harpalani et al., 1993).
The metabolic pathway of hexamethylene bisacetamide, leading to the formation of 6-acetamidohexanal and 6-acetamidohexanoic acid, has been linked to cell-differentiating activity in leukemia cells. This highlights the significance of 2,6-Diacetamidohexanoic acid and related compounds in cancer research (Subramanyam et al., 1989).
The study of competitive AMPA receptor antagonists, which are structurally similar to 2,6-Diacetamidohexanoic acid, reveals their potential in treating neurological disorders like epilepsy and Alzheimer's disease (Catarzi et al., 2007).
The simultaneous measurement of hexamethylene bisacetamide and its metabolites, including 6-acetamidohexanoic acid, via gas chromatography, is crucial in understanding the pharmacokinetics of these compounds in cancer therapy (Roth et al., 1994).
properties
IUPAC Name |
2,6-diacetamidohexanoic acid | |
---|---|---|
Source | PubChem | |
URL | https://pubchem.ncbi.nlm.nih.gov | |
Description | Data deposited in or computed by PubChem | |
InChI |
InChI=1S/C10H18N2O4/c1-7(13)11-6-4-3-5-9(10(15)16)12-8(2)14/h9H,3-6H2,1-2H3,(H,11,13)(H,12,14)(H,15,16) | |
Source | PubChem | |
URL | https://pubchem.ncbi.nlm.nih.gov | |
Description | Data deposited in or computed by PubChem | |
InChI Key |
ZHZUEHHBTYJTKY-UHFFFAOYSA-N | |
Source | PubChem | |
URL | https://pubchem.ncbi.nlm.nih.gov | |
Description | Data deposited in or computed by PubChem | |
Canonical SMILES |
CC(=O)NCCCCC(C(=O)O)NC(=O)C | |
Source | PubChem | |
URL | https://pubchem.ncbi.nlm.nih.gov | |
Description | Data deposited in or computed by PubChem | |
Molecular Formula |
C10H18N2O4 | |
Source | PubChem | |
URL | https://pubchem.ncbi.nlm.nih.gov | |
Description | Data deposited in or computed by PubChem | |
DSSTOX Substance ID |
DTXSID10956842 | |
Record name | N~2~,N~6~-Bis(1-hydroxyethylidene)lysine | |
Source | EPA DSSTox | |
URL | https://comptox.epa.gov/dashboard/DTXSID10956842 | |
Description | DSSTox provides a high quality public chemistry resource for supporting improved predictive toxicology. | |
Molecular Weight |
230.26 g/mol | |
Source | PubChem | |
URL | https://pubchem.ncbi.nlm.nih.gov | |
Description | Data deposited in or computed by PubChem | |
Product Name |
2,6-Diacetamidohexanoic acid | |
CAS RN |
35436-74-9 | |
Record name | N2,N6-Diacetyllysine | |
Source | CAS Common Chemistry | |
URL | https://commonchemistry.cas.org/detail?cas_rn=35436-74-9 | |
Description | CAS Common Chemistry is an open community resource for accessing chemical information. Nearly 500,000 chemical substances from CAS REGISTRY cover areas of community interest, including common and frequently regulated chemicals, and those relevant to high school and undergraduate chemistry classes. This chemical information, curated by our expert scientists, is provided in alignment with our mission as a division of the American Chemical Society. | |
Explanation | The data from CAS Common Chemistry is provided under a CC-BY-NC 4.0 license, unless otherwise stated. | |
Record name | N~2~,N~6~-Bis(1-hydroxyethylidene)lysine | |
Source | EPA DSSTox | |
URL | https://comptox.epa.gov/dashboard/DTXSID10956842 | |
Description | DSSTox provides a high quality public chemistry resource for supporting improved predictive toxicology. | |
Record name | 2,6-Diacetamidohexanoic acid | |
Source | FDA Global Substance Registration System (GSRS) | |
URL | https://gsrs.ncats.nih.gov/ginas/app/beta/substances/Q2F6EZ9SKB | |
Description | The FDA Global Substance Registration System (GSRS) enables the efficient and accurate exchange of information on what substances are in regulated products. Instead of relying on names, which vary across regulatory domains, countries, and regions, the GSRS knowledge base makes it possible for substances to be defined by standardized, scientific descriptions. | |
Explanation | Unless otherwise noted, the contents of the FDA website (www.fda.gov), both text and graphics, are not copyrighted. They are in the public domain and may be republished, reprinted and otherwise used freely by anyone without the need to obtain permission from FDA. Credit to the U.S. Food and Drug Administration as the source is appreciated but not required. | |
Synthesis routes and methods
Procedure details
Retrosynthesis Analysis
AI-Powered Synthesis Planning: Our tool employs the Template_relevance Pistachio, Template_relevance Bkms_metabolic, Template_relevance Pistachio_ringbreaker, Template_relevance Reaxys, Template_relevance Reaxys_biocatalysis model, leveraging a vast database of chemical reactions to predict feasible synthetic routes.
One-Step Synthesis Focus: Specifically designed for one-step synthesis, it provides concise and direct routes for your target compounds, streamlining the synthesis process.
Accurate Predictions: Utilizing the extensive PISTACHIO, BKMS_METABOLIC, PISTACHIO_RINGBREAKER, REAXYS, REAXYS_BIOCATALYSIS database, our tool offers high-accuracy predictions, reflecting the latest in chemical research and data.
Strategy Settings
Precursor scoring | Relevance Heuristic |
---|---|
Min. plausibility | 0.01 |
Model | Template_relevance |
Template Set | Pistachio/Bkms_metabolic/Pistachio_ringbreaker/Reaxys/Reaxys_biocatalysis |
Top-N result to add to graph | 6 |
Feasible Synthetic Routes
Disclaimer and Information on In-Vitro Research Products
Please be aware that all articles and product information presented on BenchChem are intended solely for informational purposes. The products available for purchase on BenchChem are specifically designed for in-vitro studies, which are conducted outside of living organisms. In-vitro studies, derived from the Latin term "in glass," involve experiments performed in controlled laboratory settings using cells or tissues. It is important to note that these products are not categorized as medicines or drugs, and they have not received approval from the FDA for the prevention, treatment, or cure of any medical condition, ailment, or disease. We must emphasize that any form of bodily introduction of these products into humans or animals is strictly prohibited by law. It is essential to adhere to these guidelines to ensure compliance with legal and ethical standards in research and experimentation.