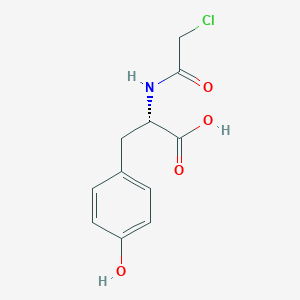
Chloroacetyl-L-tyrosine
Overview
Description
Chloroacetyl-L-tyrosine is a derivative of the amino acid L-tyrosine, where a chloroacetyl group is attached to the amino group of L-tyrosine. This compound has the molecular formula C11H12ClNO4 and a molecular weight of 257.67 g/mol . It is used in various scientific research applications due to its unique chemical properties.
Mechanism of Action
Target of Action
Chloroacetyl-L-tyrosine, also known as N-Chloroacetyl-L-tyrosine or (S)-2-(2-Chloroacetamido)-3-(4-hydroxyphenyl)propanoic acid, is a modified amino acid. It has been used as a starting point for the synthesis of macrocyclic peptides , which can target a variety of proteins and enzymes.
Mode of Action
It has been used as an initiator amino acid in the synthesis of macrocyclic peptides . These peptides can inhibit the interaction of proteins or enzymes with their substrates in an allosteric manner .
Biochemical Pathways
This compound is derived from the amino acid tyrosine. Tyrosine is produced in cells by hydroxylating the essential amino acid phenylalanine . It is a precursor for the synthesis of catecholamines, including L-DOPA, tyramine, and dopamine . Abnormal tyrosine levels in biological fluids have been linked to a number of metabolic disorders .
Pharmacokinetics
It’s known that peptides generally have the potential for small-molecule-like pharmacokinetics .
Result of Action
The macrocyclic peptides synthesized using this compound as a starting point have shown potent inhibitory effects on their target proteins or enzymes .
Action Environment
Environmental factors can influence the action, efficacy, and stability of this compound. For instance, the efficiency of post-translational modification of ribosomally-translated peptides by this compound can be influenced by the reaction conditions . Additionally, diet, which is a major environmental factor, can significantly influence the metabolism of amino acids, including tyrosine, by the gut microbiota .
Biochemical Analysis
Biochemical Properties
The chloroacetyl group attached to the L-tyrosine amino acid in Chloroacetyl-L-tyrosine serves as an electrophilic moiety . This group can be used to modify nucleophilic sites on proteins and enzymes, thereby providing insights into their function and interaction .
Molecular Mechanism
The molecular mechanism of this compound involves its electrophilic moiety interacting with nucleophilic sites on proteins and enzymes . This interaction can lead to changes in the function of these biomolecules, potentially influencing enzyme activity, protein function, and gene expression.
Preparation Methods
Synthetic Routes and Reaction Conditions
Chloroacetyl-L-tyrosine can be synthesized by reacting L-tyrosine with chloroacetyl chloride in the presence of a base. The reaction typically involves dissolving L-tyrosine in a suitable solvent, such as methanol or ethanol, and then adding chloroacetyl chloride dropwise while maintaining a low temperature to control the reaction rate . The reaction mixture is then stirred for several hours to ensure complete conversion of L-tyrosine to this compound.
Industrial Production Methods
In industrial settings, the production of this compound may involve more efficient and scalable methods. One such method includes the use of a continuous flow reactor, where L-tyrosine and chloroacetyl chloride are continuously fed into the reactor, and the product is continuously removed. This method allows for better control over reaction conditions and higher yields .
Chemical Reactions Analysis
Types of Reactions
Chloroacetyl-L-tyrosine undergoes various chemical reactions, including:
Substitution Reactions: The chloroacetyl group can be substituted with other nucleophiles, such as amines or thiols, to form new derivatives.
Oxidation and Reduction Reactions: The phenolic hydroxyl group of the tyrosine moiety can undergo oxidation to form quinones or reduction to form catechols.
Common Reagents and Conditions
Substitution Reactions: Common reagents include amines, thiols, and alcohols. These reactions are typically carried out in polar solvents like dimethyl sulfoxide (DMSO) or acetonitrile, with a base such as triethylamine to neutralize the hydrochloric acid formed during the reaction.
Oxidation Reactions: Oxidizing agents such as hydrogen peroxide or potassium permanganate are used under acidic or basic conditions.
Reduction Reactions: Reducing agents like sodium borohydride or lithium aluminum hydride are used under anhydrous conditions.
Major Products Formed
Substitution Reactions: New derivatives with different functional groups replacing the chloroacetyl group.
Oxidation Reactions: Quinones or other oxidized products.
Reduction Reactions: Catechols or other reduced products.
Scientific Research Applications
Chloroacetyl-L-tyrosine has several applications in scientific research:
Chemistry: Used as a building block for synthesizing more complex molecules and studying reaction mechanisms.
Biology: Employed in the study of enzyme-substrate interactions and protein modifications.
Medicine: Investigated for its potential use in drug development and as a precursor for bioactive compounds.
Industry: Utilized in the production of specialty chemicals and materials
Comparison with Similar Compounds
Chloroacetyl-L-tyrosine can be compared with other similar compounds, such as:
N-chloroacetyl-L-phenylalanine: Similar in structure but lacks the hydroxyl group on the aromatic ring.
N-chloroacetyl-L-serine: Contains a hydroxyl group on the side chain but lacks the aromatic ring.
N-chloroacetyl-L-cysteine: Contains a thiol group instead of a hydroxyl group
These compounds share some chemical properties but differ in their reactivity and applications, highlighting the unique features of this compound.
Properties
IUPAC Name |
2-[(2-chloroacetyl)amino]-3-(4-hydroxyphenyl)propanoic acid | |
---|---|---|
Details | Computed by Lexichem TK 2.7.0 (PubChem release 2021.05.07) | |
Source | PubChem | |
URL | https://pubchem.ncbi.nlm.nih.gov | |
Description | Data deposited in or computed by PubChem | |
InChI |
InChI=1S/C11H12ClNO4/c12-6-10(15)13-9(11(16)17)5-7-1-3-8(14)4-2-7/h1-4,9,14H,5-6H2,(H,13,15)(H,16,17) | |
Details | Computed by InChI 1.0.6 (PubChem release 2021.05.07) | |
Source | PubChem | |
URL | https://pubchem.ncbi.nlm.nih.gov | |
Description | Data deposited in or computed by PubChem | |
InChI Key |
GDOGSOZOUAVIFX-UHFFFAOYSA-N | |
Details | Computed by InChI 1.0.6 (PubChem release 2021.05.07) | |
Source | PubChem | |
URL | https://pubchem.ncbi.nlm.nih.gov | |
Description | Data deposited in or computed by PubChem | |
Canonical SMILES |
C1=CC(=CC=C1CC(C(=O)O)NC(=O)CCl)O | |
Details | Computed by OEChem 2.3.0 (PubChem release 2021.05.07) | |
Source | PubChem | |
URL | https://pubchem.ncbi.nlm.nih.gov | |
Description | Data deposited in or computed by PubChem | |
Molecular Formula |
C11H12ClNO4 | |
Details | Computed by PubChem 2.1 (PubChem release 2021.05.07) | |
Source | PubChem | |
URL | https://pubchem.ncbi.nlm.nih.gov | |
Description | Data deposited in or computed by PubChem | |
Molecular Weight |
257.67 g/mol | |
Details | Computed by PubChem 2.1 (PubChem release 2021.05.07) | |
Source | PubChem | |
URL | https://pubchem.ncbi.nlm.nih.gov | |
Description | Data deposited in or computed by PubChem | |
CAS No. |
1145-56-8 | |
Record name | N-Chloroacetyl-L-tyrosine | |
Source | ChemIDplus | |
URL | https://pubchem.ncbi.nlm.nih.gov/substance/?source=chemidplus&sourceid=0001145568 | |
Description | ChemIDplus is a free, web search system that provides access to the structure and nomenclature authority files used for the identification of chemical substances cited in National Library of Medicine (NLM) databases, including the TOXNET system. | |
Record name | NSC523824 | |
Source | DTP/NCI | |
URL | https://dtp.cancer.gov/dtpstandard/servlet/dwindex?searchtype=NSC&outputformat=html&searchlist=523824 | |
Description | The NCI Development Therapeutics Program (DTP) provides services and resources to the academic and private-sector research communities worldwide to facilitate the discovery and development of new cancer therapeutic agents. | |
Explanation | Unless otherwise indicated, all text within NCI products is free of copyright and may be reused without our permission. Credit the National Cancer Institute as the source. | |
Retrosynthesis Analysis
AI-Powered Synthesis Planning: Our tool employs the Template_relevance Pistachio, Template_relevance Bkms_metabolic, Template_relevance Pistachio_ringbreaker, Template_relevance Reaxys, Template_relevance Reaxys_biocatalysis model, leveraging a vast database of chemical reactions to predict feasible synthetic routes.
One-Step Synthesis Focus: Specifically designed for one-step synthesis, it provides concise and direct routes for your target compounds, streamlining the synthesis process.
Accurate Predictions: Utilizing the extensive PISTACHIO, BKMS_METABOLIC, PISTACHIO_RINGBREAKER, REAXYS, REAXYS_BIOCATALYSIS database, our tool offers high-accuracy predictions, reflecting the latest in chemical research and data.
Strategy Settings
Precursor scoring | Relevance Heuristic |
---|---|
Min. plausibility | 0.01 |
Model | Template_relevance |
Template Set | Pistachio/Bkms_metabolic/Pistachio_ringbreaker/Reaxys/Reaxys_biocatalysis |
Top-N result to add to graph | 6 |
Feasible Synthetic Routes
Q1: What is the significance of the secondary peptide bond in substrates for Carboxypeptidase, and how does Chloroacetyl-L-tyrosine relate to this?
A1: Research indicates that the presence and proximity of a secondary peptide bond significantly influence the susceptibility of substrates to hydrolysis by Carboxypeptidase []. While typical peptide substrates for this enzyme possess a secondary peptide bond separated from the hydrolyzable bond by one methylene group, this compound lacks this feature. Despite this absence, this compound, alongside its phenylalanine analogue, has been recognized as a substrate for Carboxypeptidase []. This observation suggests that while the secondary peptide bond plays a role in substrate recognition and binding, it is not an absolute requirement for hydrolysis by Carboxypeptidase.
Q2: Can you elaborate on the use of this compound in studying Carboxypeptidase activity?
A2: this compound holds historical significance as one of the earliest substrates employed to assess the enzymatic activity of Carboxypeptidase []. While initially utilized with less purified enzyme preparations, its application extends to kinetic studies with the crystalline enzyme []. These investigations, often focusing on the kinetics of hydrolysis, aim to unravel the enzyme's mechanism of action and specificity.
Q3: Beyond Carboxypeptidase, has this compound been identified as a substrate for other proteases?
A3: Yes, research has shown that a protease isolated from the fungus Mortierella renispora Dixon-Stewart exhibits the ability to hydrolyze this compound []. This protease, characterized by its cathodic movement during isolation, showcases a broader substrate specificity, also acting upon substrates like N-carbobenzoxy-α-L-glutamyl-DL-alanine []. This finding highlights the potential of this compound as a tool for investigating the activity and specificity of diverse proteolytic enzymes.
Q4: How does the stability of the protease from Mortierella renispora Dixon-Stewart, which hydrolyzes this compound, compare to other proteases from the same source?
A4: Studies have revealed the presence of two distinct proteases in the culture medium of Mortierella renispora Dixon-Stewart, each displaying unique properties []. Notably, the protease exhibiting activity against this compound, identified as the cathodic component based on its electrophoretic mobility, demonstrates greater stability at 58°C compared to the anodic component []. This difference in heat stability suggests variations in their structural conformation and potential applications under different temperature conditions.
Disclaimer and Information on In-Vitro Research Products
Please be aware that all articles and product information presented on BenchChem are intended solely for informational purposes. The products available for purchase on BenchChem are specifically designed for in-vitro studies, which are conducted outside of living organisms. In-vitro studies, derived from the Latin term "in glass," involve experiments performed in controlled laboratory settings using cells or tissues. It is important to note that these products are not categorized as medicines or drugs, and they have not received approval from the FDA for the prevention, treatment, or cure of any medical condition, ailment, or disease. We must emphasize that any form of bodily introduction of these products into humans or animals is strictly prohibited by law. It is essential to adhere to these guidelines to ensure compliance with legal and ethical standards in research and experimentation.