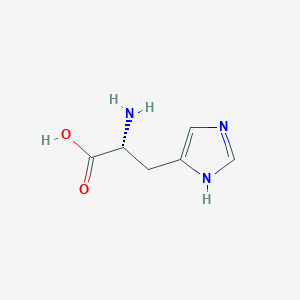
D-Histidine
Overview
Description
D-histidine is an enantiomer of the amino acid histidine, which is essential for protein synthesis and various metabolic processes in living organisms. Unlike its more common counterpart, L-histidine, this compound is less prevalent in nature but has unique properties and applications in scientific research and industry.
Mechanism of Action
Target of Action
D-Histidine primarily targets Pseudomonas aeruginosa , an opportunistic gram-negative pathogenic microorganism . It interacts with the organism’s virulence and quorum sensing (QS)-associated genes . This compound has also been identified as a prospective anti-biofilm agent .
Mode of Action
This compound downregulates the mRNA expression of virulence and QS-associated genes in Pseudomonas aeruginosa PAO1 without affecting bacterial growth . It significantly reduces the motility and pathogenicity of PAO1 . This compound has been shown to inhibit biofilm formation and trigger the disassembly of mature biofilms . It increases the susceptibility of PAO1 to amikacin, an antibiotic .
Biochemical Pathways
Histidine, the L-form of this compound, is synthesized by most organisms, including bacteria, archaea, some lower eukaryotes, and plants . Histidine-producing microorganisms can synthesize this amino acid using a common biosynthetic pathway, which consists of ten biochemical steps . This compound can be converted to 3-methylhistidine, which serves as a biomarker for skeletal muscle damage, by certain methyltransferase enzymes .
Pharmacokinetics
Covalent drugs and chemical probes, which could include this compound, often possess pharmacological advantages over reversible binding ligands, such as enhanced potency and pharmacodynamic duration .
Result of Action
This compound combats Pseudomonas aeruginosa by reducing biofilm formation and increasing biofilm disassembly . The combination of amikacin and this compound induces a synergistic effect against Pseudomonas aeruginosa biofilms . This suggests the potential utility of this compound as a preventive strategy against biofilm-associated infections caused by Pseudomonas aeruginosa .
Action Environment
The action of this compound can be influenced by environmental factors. For instance, high concentrations of this compound inhibit biofilm formation, disperse already generated biofilms, and enhance the antibacterial effect of amikacin . The growth inhibition of pseudomonas aeruginosa by this compound is observed, especially at high concentrations .
Biochemical Analysis
Biochemical Properties
D-Histidine, like its L-isomer, contains an α-amino group, a carboxylic acid group, and an imidazole side chain, classifying it as a positively charged amino acid at physiological pH . The imidazole side chain can donate and accept protons, thus enabling this compound to participate in various enzymatic reactions .
Cellular Effects
This compound has been shown to have significant effects on various types of cells and cellular processes. For instance, it has been found to downregulate the mRNA expression of virulence and quorum sensing (QS)-associated genes in Pseudomonas aeruginosa PAO1 without affecting bacterial growth . Moreover, this compound has been shown to inhibit biofilm formation and trigger the disassembly of mature biofilms .
Molecular Mechanism
The molecular mechanism of this compound involves its interaction with various biomolecules. For instance, this compound has been shown to protect against zinc-induced neurotoxicity not by inhibiting zinc chelation, thereby preventing increases in intracellular Zn2+ . Furthermore, this compound is metabolized to the amino acid glutamate through a series of intermediate molecules in a pathway that requires the enzyme histidine ammonia lyase (HAL) .
Temporal Effects in Laboratory Settings
In laboratory settings, this compound has been shown to have temporal effects on the cells it interacts with. For instance, this compound has been shown to combat biofilm formation and enhance the effect of amikacin against Pseudomonas aeruginosa in vitro .
Dosage Effects in Animal Models
The effects of this compound vary with different dosages in animal models. For instance, a diet rich in the amino acid histidine has been shown to increase the effectiveness of methotrexate treatment and lower toxicity in mice .
Metabolic Pathways
This compound is involved in various metabolic pathways. It is metabolized to the amino acid glutamate through a series of intermediate molecules in a pathway that requires the enzyme histidine ammonia lyase (HAL) .
Transport and Distribution
This compound is transported and distributed within cells and tissues. The oligopeptide/histidine transporters PHT1 and PHT2, two mammalian solute carrier family 15A proteins, mediate the transmembrane transport of histidine and some di/tripeptides via proton gradient .
Subcellular Localization
This compound and its related proteins have been shown to localize in various subcellular compartments. This localization is lost when the histidine repeat is deleted .
Preparation Methods
Synthetic Routes and Reaction Conditions: D-histidine can be synthesized through several methods, including:
Enzymatic Resolution: This method involves the use of enzymes to selectively convert L-histidine to this compound.
Chemical Synthesis: This involves the use of chemical reagents to convert L-histidine to this compound through a series of reactions, including racemization and resolution steps.
Industrial Production Methods: Industrial production of this compound typically involves fermentation processes using genetically modified microorganisms. These microorganisms are engineered to produce this compound in large quantities, which is then purified through various chromatographic techniques.
Chemical Reactions Analysis
Types of Reactions: D-histidine undergoes several types of chemical reactions, including:
Oxidation: this compound can be oxidized to form various products, including imidazole derivatives.
Reduction: Reduction reactions can convert this compound to its corresponding alcohols or amines.
Substitution: this compound can undergo substitution reactions, where functional groups on the imidazole ring are replaced with other groups.
Common Reagents and Conditions:
Oxidation: Common oxidizing agents include hydrogen peroxide and potassium permanganate.
Reduction: Reducing agents such as sodium borohydride and lithium aluminum hydride are commonly used.
Substitution: Substitution reactions often involve halogenating agents like bromine or chlorine.
Major Products:
Oxidation Products: Imidazole derivatives.
Reduction Products: Alcohols and amines.
Substitution Products: Halogenated histidine derivatives.
Scientific Research Applications
D-histidine has a wide range of applications in scientific research, including:
Chemistry: Used as a chiral building block in the synthesis of complex molecules.
Biology: Studied for its role in enzyme catalysis and protein interactions.
Industry: Used in the production of pharmaceuticals and as a food additive.
Comparison with Similar Compounds
L-histidine: The more common enantiomer, essential for protein synthesis.
DL-histidine: A racemic mixture of D-histidine and L-histidine.
Comparison:
Applications: While L-histidine is widely used in nutritional supplements and medical treatments, this compound’s applications are more specialized, particularly in research and industrial settings.
Properties
IUPAC Name |
(2R)-2-amino-3-(1H-imidazol-5-yl)propanoic acid | |
---|---|---|
Source | PubChem | |
URL | https://pubchem.ncbi.nlm.nih.gov | |
Description | Data deposited in or computed by PubChem | |
InChI |
InChI=1S/C6H9N3O2/c7-5(6(10)11)1-4-2-8-3-9-4/h2-3,5H,1,7H2,(H,8,9)(H,10,11)/t5-/m1/s1 | |
Source | PubChem | |
URL | https://pubchem.ncbi.nlm.nih.gov | |
Description | Data deposited in or computed by PubChem | |
InChI Key |
HNDVDQJCIGZPNO-RXMQYKEDSA-N | |
Source | PubChem | |
URL | https://pubchem.ncbi.nlm.nih.gov | |
Description | Data deposited in or computed by PubChem | |
Canonical SMILES |
C1=C(NC=N1)CC(C(=O)O)N | |
Source | PubChem | |
URL | https://pubchem.ncbi.nlm.nih.gov | |
Description | Data deposited in or computed by PubChem | |
Isomeric SMILES |
C1=C(NC=N1)C[C@H](C(=O)O)N | |
Source | PubChem | |
URL | https://pubchem.ncbi.nlm.nih.gov | |
Description | Data deposited in or computed by PubChem | |
Molecular Formula |
C6H9N3O2 | |
Source | PubChem | |
URL | https://pubchem.ncbi.nlm.nih.gov | |
Description | Data deposited in or computed by PubChem | |
DSSTOX Substance ID |
DTXSID70889344 | |
Record name | D-Histidine | |
Source | EPA DSSTox | |
URL | https://comptox.epa.gov/dashboard/DTXSID70889344 | |
Description | DSSTox provides a high quality public chemistry resource for supporting improved predictive toxicology. | |
Molecular Weight |
155.15 g/mol | |
Source | PubChem | |
URL | https://pubchem.ncbi.nlm.nih.gov | |
Description | Data deposited in or computed by PubChem | |
CAS No. |
351-50-8 | |
Record name | D-Histidine | |
Source | CAS Common Chemistry | |
URL | https://commonchemistry.cas.org/detail?cas_rn=351-50-8 | |
Description | CAS Common Chemistry is an open community resource for accessing chemical information. Nearly 500,000 chemical substances from CAS REGISTRY cover areas of community interest, including common and frequently regulated chemicals, and those relevant to high school and undergraduate chemistry classes. This chemical information, curated by our expert scientists, is provided in alignment with our mission as a division of the American Chemical Society. | |
Explanation | The data from CAS Common Chemistry is provided under a CC-BY-NC 4.0 license, unless otherwise stated. | |
Record name | D-Histidine | |
Source | ChemIDplus | |
URL | https://pubchem.ncbi.nlm.nih.gov/substance/?source=chemidplus&sourceid=0000351508 | |
Description | ChemIDplus is a free, web search system that provides access to the structure and nomenclature authority files used for the identification of chemical substances cited in National Library of Medicine (NLM) databases, including the TOXNET system. | |
Record name | D-Histidine | |
Source | EPA Chemicals under the TSCA | |
URL | https://www.epa.gov/chemicals-under-tsca | |
Description | EPA Chemicals under the Toxic Substances Control Act (TSCA) collection contains information on chemicals and their regulations under TSCA, including non-confidential content from the TSCA Chemical Substance Inventory and Chemical Data Reporting. | |
Record name | D-Histidine | |
Source | EPA DSSTox | |
URL | https://comptox.epa.gov/dashboard/DTXSID70889344 | |
Description | DSSTox provides a high quality public chemistry resource for supporting improved predictive toxicology. | |
Record name | D-histidine hydrochloride monohydrate | |
Source | European Chemicals Agency (ECHA) | |
URL | https://echa.europa.eu/substance-information/-/substanceinfo/100.005.922 | |
Description | The European Chemicals Agency (ECHA) is an agency of the European Union which is the driving force among regulatory authorities in implementing the EU's groundbreaking chemicals legislation for the benefit of human health and the environment as well as for innovation and competitiveness. | |
Explanation | Use of the information, documents and data from the ECHA website is subject to the terms and conditions of this Legal Notice, and subject to other binding limitations provided for under applicable law, the information, documents and data made available on the ECHA website may be reproduced, distributed and/or used, totally or in part, for non-commercial purposes provided that ECHA is acknowledged as the source: "Source: European Chemicals Agency, http://echa.europa.eu/". Such acknowledgement must be included in each copy of the material. ECHA permits and encourages organisations and individuals to create links to the ECHA website under the following cumulative conditions: Links can only be made to webpages that provide a link to the Legal Notice page. | |
Record name | D-HISTIDINE | |
Source | FDA Global Substance Registration System (GSRS) | |
URL | https://gsrs.ncats.nih.gov/ginas/app/beta/substances/4JE5HRM5CH | |
Description | The FDA Global Substance Registration System (GSRS) enables the efficient and accurate exchange of information on what substances are in regulated products. Instead of relying on names, which vary across regulatory domains, countries, and regions, the GSRS knowledge base makes it possible for substances to be defined by standardized, scientific descriptions. | |
Explanation | Unless otherwise noted, the contents of the FDA website (www.fda.gov), both text and graphics, are not copyrighted. They are in the public domain and may be republished, reprinted and otherwise used freely by anyone without the need to obtain permission from FDA. Credit to the U.S. Food and Drug Administration as the source is appreciated but not required. | |
Retrosynthesis Analysis
AI-Powered Synthesis Planning: Our tool employs the Template_relevance Pistachio, Template_relevance Bkms_metabolic, Template_relevance Pistachio_ringbreaker, Template_relevance Reaxys, Template_relevance Reaxys_biocatalysis model, leveraging a vast database of chemical reactions to predict feasible synthetic routes.
One-Step Synthesis Focus: Specifically designed for one-step synthesis, it provides concise and direct routes for your target compounds, streamlining the synthesis process.
Accurate Predictions: Utilizing the extensive PISTACHIO, BKMS_METABOLIC, PISTACHIO_RINGBREAKER, REAXYS, REAXYS_BIOCATALYSIS database, our tool offers high-accuracy predictions, reflecting the latest in chemical research and data.
Strategy Settings
Precursor scoring | Relevance Heuristic |
---|---|
Min. plausibility | 0.01 |
Model | Template_relevance |
Template Set | Pistachio/Bkms_metabolic/Pistachio_ringbreaker/Reaxys/Reaxys_biocatalysis |
Top-N result to add to graph | 6 |
Feasible Synthetic Routes
Q1: Does D-Histidine exhibit any biological activity?
A1: Yes, research suggests that this compound possesses various biological activities, including anti-inflammatory [] and appetite-suppressing effects []. Additionally, it shows potential in inhibiting the growth of specific bacteria like Porphyromonas gingivalis [, ].
Q2: How does this compound exert its anti-inflammatory effect?
A2: Studies indicate that this compound, similar to L-Histidine, can scavenge reactive oxygen species (ROS) []. This ROS scavenging ability is attributed to the presence of the imidazole ring in its structure.
Q3: Can this compound cross the blood-brain barrier?
A3: Yes, research has shown that this compound can penetrate the blood-brain barrier, although at a slower rate compared to L-Histidine []. Interestingly, once in the brain, this compound is removed very slowly, leading to its continuous accumulation [].
Q4: Does this compound influence histamine levels in the brain?
A4: While L-Histidine is a precursor to histamine, studies on rats indicate that this compound administration does not affect brain histamine concentrations []. This suggests that this compound might exert its appetite-suppressing effects through a different pathway than L-Histidine [].
Q5: Can bacteria utilize this compound?
A5: Yes, certain bacteria, including Salmonella typhimurium [, , , ] and Fusobacterium nucleatum [], can utilize this compound. This utilization often involves the conversion of this compound to L-Histidine through racemization [, , , ].
Q6: Are there specific transport systems for this compound in bacteria?
A6: Research on Salmonella typhimurium suggests that mutations in genes encoding histidine permease can allow for this compound transport, although at low levels []. These findings indicate that certain bacterial transporters might have a low affinity for this compound.
Q7: What is the molecular formula and weight of this compound?
A7: The molecular formula of this compound is C6H9N3O2, and its molecular weight is 155.15 g/mol.
Q8: What spectroscopic techniques are useful for characterizing this compound?
A8: Various spectroscopic methods are used to characterize this compound, including:
- NMR Spectroscopy: 1H and 13C NMR can provide structural information and are particularly useful for studying the interaction of this compound with metal complexes and nucleotides [].
- Circular Dichroism (CD) Spectroscopy: This technique can differentiate between enantiomers and is used to study the chiral recognition properties of this compound and its complexes [, , ].
- Terahertz Spectroscopy: This method has been employed to detect low content enantiomer impurities in L-Histidine crystals, demonstrating its sensitivity in differentiating between L- and this compound [].
Q9: Is this compound stable under different conditions?
A9: While specific studies on the stability of this compound are limited in the provided research, it is known that amino acids, in general, can degrade under certain conditions. Factors like temperature, pH, and the presence of other chemicals can influence their stability. Further research is needed to fully understand the stability profile of this compound.
Q10: Are there any applications of this compound related to material compatibility?
A10: Research shows the potential application of this compound in chiral modification of zeolitic imidazolate framework-90 (ZIF-90) []. This modified material demonstrated enantioselectivity in separating various drug enantiomers, highlighting the potential of this compound in developing chiral stationary phases for analytical separations [].
Q11: Have computational methods been used to study this compound?
A11: Yes, computational chemistry techniques have been employed to investigate this compound:
- Molecular Modeling: Molecular modeling studies have provided insights into the interaction of this compound with fullerene derivatives, supporting the development of enantioselective sensors [].
Q12: Are there any in vivo studies demonstrating the efficacy of this compound?
A15: Yes, this compound has demonstrated promising neuroprotective effects in an in vivo rat model of zinc-induced neurotoxicity, suggesting its potential therapeutic application in conditions like vascular-type dementia [].
Disclaimer and Information on In-Vitro Research Products
Please be aware that all articles and product information presented on BenchChem are intended solely for informational purposes. The products available for purchase on BenchChem are specifically designed for in-vitro studies, which are conducted outside of living organisms. In-vitro studies, derived from the Latin term "in glass," involve experiments performed in controlled laboratory settings using cells or tissues. It is important to note that these products are not categorized as medicines or drugs, and they have not received approval from the FDA for the prevention, treatment, or cure of any medical condition, ailment, or disease. We must emphasize that any form of bodily introduction of these products into humans or animals is strictly prohibited by law. It is essential to adhere to these guidelines to ensure compliance with legal and ethical standards in research and experimentation.