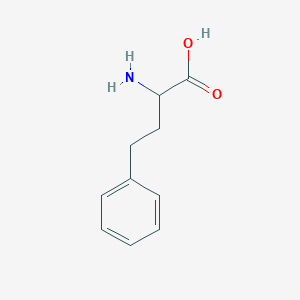
DL-Homophenylalanine
Overview
Description
DL-Homophenylalanine (CAS: 1012-05-1) is a synthetic amino acid derivative with the molecular formula C₁₀H₁₃NO₂ and a molecular weight of 179.22 g/mol . Structurally, it is a homolog of phenylalanine, featuring an additional methylene group (–CH₂–) in its side chain, resulting in the systematic name (±)-2-amino-4-phenylbutyric acid . Key properties include:
Scientific Research Applications
Chemical Synthesis
Chiral Building Block
DL-Homophenylalanine serves as a chiral building block in the synthesis of complex molecules. Its structural similarity to phenylalanine allows for the incorporation into peptide sequences, enabling modifications that can enhance peptide activity and stability. For instance, studies have shown that substituting this compound in the N-terminal region of peptides can significantly alter their biological functions.
Synthesis of Pharmaceuticals
This compound is crucial in the pharmaceutical industry for the synthesis of angiotensin-converting enzyme inhibitors, such as Enalapril and Lisinopril. The ability to produce optically active derivatives through biocatalytic methods enhances its utility in drug development .
Biological Applications
Enzyme Inhibition
Research indicates that derivatives of this compound can act as selective inhibitors for various enzymes, including dipeptidyl peptidase IV (DPP-IV), which is vital for managing type 2 diabetes. Modifications to its structure have led to the development of potent inhibitors with improved pharmacokinetic properties .
Biosynthetic Pathways
Studies have identified biosynthetic genes associated with homophenylalanine, highlighting its role as an intermediate in the synthesis of benzyl glucosinolate. This underscores its importance in plant metabolism and potential applications in agricultural biotechnology .
Agricultural Biotechnology
Plant Growth Regulation
this compound has been investigated for its effects on plant growth and organogenesis. Research shows it influences photosynthetic pigment profiles and foliar structures in micropropagated plants, such as bananas. Its application in tissue culture has demonstrated enhanced growth rates and organ formation .
Biocatalysis
Biocatalytic Processes
The compound is significant in biocatalysis, where biological systems facilitate chemical transformations. Studies have utilized recombinant Escherichia coli to produce this compound through enzymatic processes, showcasing its potential as a sustainable method for synthesizing pharmaceuticals .
Data Tables
Application Area | Description | Case Study/Example |
---|---|---|
Chemical Synthesis | Chiral building block for complex molecules | Modification of peptide sequences for enhanced stability |
Pharmaceutical Production | Precursor for ACE inhibitors like Enalapril | Enantioselective synthesis methods developed |
Enzyme Inhibition | Selective inhibitors for DPP-IV | Development of beta-homophenylalanine derivatives |
Plant Biotechnology | Enhances growth and organogenesis in plants | Studies on micropropagated banana plants |
Biocatalysis | Sustainable production methods using recombinant bacteria | Use of E. coli for this compound synthesis |
Mechanism of Action
The mechanism by which Rac-2-amino-4-phenylbutanoic acid exerts its effects involves its interaction with specific enzymes and receptors in the body. For example, it can act as a substrate for phenylalanine dehydrogenase, leading to the production of other biologically active compounds . The molecular targets and pathways involved include the inhibition of angiotensin-converting enzyme, which plays a role in regulating blood pressure .
Comparison with Similar Compounds
Structural and Functional Comparison with Similar Compounds
Structural Analogues
DL-Homophenylalanine belongs to a family of phenylalanine derivatives. Below is a comparison with structurally related compounds:
Enzymatic Interactions
Phenylalanine Dehydrogenase (PDH)
- L-Phenylalanine : High substrate specificity (86.2 U/mg activity in deamination) .
- This compound : Inert at 4 mM concentration .
- D-Phenylalanine, DL-Phenylglycine, DL-Tert-leucine : Also inert, highlighting the enzyme’s strict requirement for side-chain length and stereochemistry .
CYP79A2 (Arabidopsis thaliana)
- L-Phenylalanine: Converted to phenylacetaldoxime (biosynthesis of glucosinolates) .
- This compound: Not metabolized despite structural similarity, indicating steric or electronic incompatibility .
- L-Tyrosine, L-Tryptophan : Similarly inert, underscoring CYP79A2’s narrow substrate range .
Physicochemical Properties
Key Research Findings
Enzyme Substrate Specificity :
- The extended side chain of this compound prevents recognition by enzymes like PDH and CYP79A2, which evolved to process L-phenylalanine’s shorter side chain .
- Substituting the β-carbon with a methyl group (e.g., DL-tert-leucine) also disrupts enzyme binding .
Biochemical Applications: this compound serves as a non-metabolizable analog in studies of phenylalanine transport or enzyme inhibition . Its stability at high temperatures (melting point ≥282°C) makes it suitable for harsh reaction conditions .
Commercial Relevance: Priced at ¥9,000–108,000 per 1–25 g (), it is costlier than natural amino acids due to synthetic complexity.
Biological Activity
DL-Homophenylalanine is a non-proteinogenic amino acid with the molecular formula CHNO and a molecular weight of 179.22 g/mol. It is characterized by a 2-phenylethyl side chain, distinguishing it from its natural counterpart, phenylalanine, which contains a benzyl group. This compound is utilized in various biochemical and pharmaceutical applications due to its structural properties and potential biological activities.
1. Peptide Synthesis and Modifications
This compound's structural similarity to phenylalanine allows it to be incorporated into peptide sequences, enabling researchers to modify peptide properties and functionalities. This capability is particularly useful in studying the impact of specific amino acid substitutions on peptide activity and stability. For example, modifications of the N-terminal region of arginine vasopressin using this compound have provided insights into the hormone's functional roles.
2. Enzyme Inhibition Potential
Research has demonstrated that derivatives of this compound can serve as enzyme inhibitors. By altering its chemical structure, scientists have developed compounds that selectively inhibit enzymes such as dipeptidyl peptidase IV (DPP-IV), which plays a significant role in type 2 diabetes management. These findings suggest that this compound derivatives could be promising candidates for novel therapeutic agents.
3. Plant Growth and Development
In plant research, this compound has been investigated for its effects on growth and organogenesis. Studies have shown that it influences photosynthetic pigment profiles and foliar structures in micropropagated plants, such as bananas. Additionally, its role in organogenesis within plant cotyledons has been explored, indicating its potential utility in agricultural biotechnology.
4. Biocatalysis Applications
This compound is also significant in biocatalysis, where biological systems are used for chemical transformations. Research has focused on using recombinant Escherichia coli cells to convert this compound into its L-enantiomer, which is more biologically relevant. This biocatalytic approach offers a sustainable method for producing L-homophenylalanine for various applications .
Comparative Analysis with Related Compounds
The following table summarizes the structural features and unique properties of this compound compared to related compounds:
Compound Name | Structural Features | Unique Properties |
---|---|---|
L-Homophenylalanine | Non-proteinogenic amino acid | Involved in neurotransmitter synthesis |
D-Homophenylalanine | Optical isomer of homophenylalanine | Primarily used in pharmaceutical applications |
Phenylalanine | Standard proteinogenic amino acid | Precursor for neurotransmitters like dopamine |
2-Amino-4-phenylbutyric Acid | Structural analogue | Potentially different biological activities |
Case Study 1: Enzyme Inhibition
A study investigated the inhibitory effects of beta-homophenylalanine derivatives on DPP-IV. The derivatives demonstrated potent inhibition, suggesting their potential as therapeutic agents for managing type 2 diabetes.
Case Study 2: Plant Growth Regulation
Research conducted on the application of this compound in micropropagation revealed significant enhancements in photosynthetic efficiency and biomass accumulation in banana plants. The study highlighted its role in promoting growth through modulation of metabolic pathways related to chlorophyll synthesis.
Chemical Reactions Analysis
Oxidation Reactions
DL-HPA undergoes oxidation at the α-carbon or side chain, yielding keto acids or hydroxylated derivatives.
Key Reagents and Conditions:
- Potassium permanganate (KMnO₄) or chromium trioxide (CrO₃) under acidic conditions oxidize the amino group or phenyl side chain.
- Enzymatic oxidation via phenylalanine dehydrogenase converts DL-HPA to 2-oxo-4-phenylbutanoic acid, a precursor in asymmetric synthesis .
Outcomes:
- Oxidized derivatives serve as intermediates for peptide modifications or enzyme inhibitors (e.g., DPP-IV inhibitors for diabetes).
Reduction Reactions
Reduction of DL-HPA or its precursors enables access to chiral amino alcohols.
Key Methods:
- Chemical Reduction: Sodium borohydride (NaBH₄) or lithium aluminum hydride (LiAlH₄) reduces keto intermediates (e.g., 2-oxo-4-phenylbutanoate) to DL-HPA with moderate enantioselectivity.
- Biocatalytic Reduction: Carbonyl reductases (e.g., from E. coli) achieve enantiopure L-HPA (>99% ee) via asymmetric reduction of ethyl 2-oxo-4-phenylbutanoate .
Table 1: Comparison of Reduction Methods
Method | Catalyst/Reagent | Yield (%) | Enantiomeric Excess (ee) | Source |
---|---|---|---|---|
Chemical (NaBH₄) | - | 65–75 | 50–60% (racemic) | |
Biocatalytic (TipheDH) | Engineered dehydrogenase | 94 | >99% (L-HPA) |
Substitution Reactions
The amino or carboxyl groups of DL-HPA participate in nucleophilic substitutions, enabling side-chain diversification.
Examples:
- Halogenation: Reaction with thionyl chloride (SOCl₂) or PCl₅ converts the carboxyl group to acyl chlorides for peptide coupling .
- Enzymatic Transamination: Transaminases replace the amino group with keto functionalities, generating non-natural amino acids .
Case Study:
- Substitution of DL-HPA’s phenyl group with fluorinated analogs (e.g., m-fluorophenylalanine) via microbial pathways yields bioactive derivatives .
Pd-Catalyzed Tandem Reactions
Palladium-mediated C–H functionalization offers efficient routes to DL-HPA precursors.
Key Process:
- Sequential C–H Amination/Arylation: Pd(II)/sulfoxide catalysts enable one-pot synthesis of α- and β-homophenylalanine derivatives from α-olefins and aryl boronic acids .
Conditions:
- Catalyst: 10 mol% Pd(OAc)₂ with sulfoxide ligands.
- Reagents: N-nosylcarbamates for amination; aryl boronic acids for arylation.
- Yield: 79–85% with >20:1 regioselectivity .
Table 2: Pd-Catalyzed Reaction Scope
Starting Material | Product | Yield (%) | Selectivity (E:Z) |
---|---|---|---|
3-Butenol | β-Homophenylalanine | 79 | >20:1 |
4-Pentenol | α-Homophenylalanine | 82 | >20:1 |
Peptide Incorporation
DL-HPA’s structural similarity to phenylalanine allows its integration into peptides, altering pharmacokinetic properties.
Applications:
Q & A
Basic Research Questions
Q. What are the standard methods for synthesizing DL-Homophenylalanine with high enantiomeric purity?
this compound (±-2-Amino-4-phenylbutyric acid) is typically synthesized via Strecker synthesis or enzymatic resolution. Key steps include:
- Chiral resolution : Use of chiral auxiliaries or chromatography (e.g., HPLC with chiral stationary phases) to separate enantiomers, as commercial products report ≥95% purity .
- Crystallization : Recrystallization in polar solvents (e.g., ethanol/water mixtures) to achieve a melting point of 282°C, confirming purity .
- Quality control : NMR and mass spectrometry (F.W. 179.22) for structural validation .
Q. How can researchers validate the purity of this compound in experimental settings?
- Analytical chromatography : Reverse-phase HPLC with UV detection (λ = 254 nm) to assess purity ≥95% .
- Thermal analysis : Differential scanning calorimetry (DSC) to verify the sharp melting point at 282°C .
- Elemental analysis : Confirm molecular composition (C10H13NO2) via combustion analysis .
Q. What role does this compound play in studying amino acid transport mechanisms?
- Competitive inhibition assays : this compound is used to probe substrate specificity of amino acid transporters (e.g., LAT1 or ASCT2) by comparing uptake rates with natural substrates like phenylalanine .
- Radiolabeling : Tritiated or 14C-labeled this compound can track transport kinetics in cell cultures .
Advanced Research Questions
Q. How does this compound’s inertness in phenylalanine dehydrogenase reactions inform substrate specificity models?
- Enzyme kinetics : this compound shows no activity (<1% relative to L-phenylalanine) in Thermophilic bacterial phenylalanine dehydrogenase assays, suggesting strict stereochemical and side-chain length requirements for substrate recognition .
- Structural modeling : Molecular docking simulations reveal that the extended side chain (4-phenylbutyric acid vs. 3-phenylpropionic acid in phenylalanine) disrupts binding to the active site .
Q. What contradictory findings exist regarding this compound’s metabolic pathways in bacterial systems?
- CYP79A2 paradox : While CYP79A2 from Arabidopsis thaliana metabolizes L-phenylalanine to phenylacetaldoxime, it fails to catalyze this compound oxidation, indicating substrate specificity barriers in cytochrome P450 enzymes .
- Resolution : Cross-species enzyme assays (e.g., testing bacterial vs. plant P450s) and isotopic tracing (e.g., 13C-DL-Homophenylalanine) can clarify metabolic inertness .
Q. How can researchers optimize this compound’s use in peptide mimetics for drug discovery?
- Solid-phase peptide synthesis (SPPS) : Incorporate this compound as a non-natural amino acid to enhance protease resistance. Use Fmoc-protected derivatives (e.g., H-DL-Hph-OH) with coupling reagents like HBTU .
- Conformational analysis : Circular dichroism (CD) and X-ray crystallography to study backbone flexibility and phenyl group interactions in peptide chains .
Q. What experimental designs address discrepancies in this compound’s solubility across studies?
- Solvent screening : Test solubility in buffered aqueous solutions (pH 2–9) and organic solvents (DMSO, DMF) at 25°C. Note: Low solubility in water (<1 mg/mL) necessitates DMSO for stock solutions .
- Controlled conditions : Standardize temperature (e.g., 0–6°C storage to prevent degradation) and ionic strength to ensure reproducibility .
Q. Methodological Guidance for Data Contradictions
Q. How should researchers analyze conflicting data on this compound’s enzyme inhibition profiles?
- Systematic review : Compare assay conditions (e.g., pH, cofactors like NAD+) across studies. For example, NADP (NADPH) inertness in some dehydrogenase assays may explain variability .
- Meta-analysis : Aggregate kinetic parameters (Km, Vmax) from published datasets to identify outliers or methodological biases .
Q. What strategies validate this compound’s role in metabolic pathway engineering?
Preparation Methods
Chemical Synthesis of DL-Homophenylalanine
Strecker Synthesis and Reductive Amination
The Strecker synthesis remains a foundational route for racemic amino acids. For DL-HPA, this involves reacting 2-oxo-4-phenylbutyric acid (OPBA) with ammonium cyanide, followed by hydrolysis to yield the racemic amino acid . OPBA itself is synthesized via oxidation of hydrocinnamic acid (3-phenylpropanoic acid) using methods such as Friedel-Crafts acylation or microbial oxidation . Reductive amination offers an alternative, where OPBA is treated with ammonia and a reducing agent like sodium cyanoborohydride under mild acidic conditions. This method avoids cyanide use but requires precise pH control to prevent side reactions .
Hydrolysis of Racemic Esters
DL-HPA ethyl ester (DL-HPAE), a precursor, is synthesized through esterification of OPBA with ethanol, followed by reductive amination. Hydrolysis of DL-HPAE under acidic or alkaline conditions yields DL-HPA. For instance, US5552317A demonstrates that refluxing DL-HPAE with hydrochloric acid achieves near-quantitative conversion . This method benefits from the ester’s stability during storage and transport, though hydrolysis necessitates careful neutralization to isolate the free amino acid .
Enzymatic Resolution and Dynamic Kinetic Processes
Lipase-Catalyzed Ester Hydrolysis
Enzymatic resolution leverages lipases or acylases to enantioselectively hydrolyze DL-HPAE. For example, lipase from Candida rugosa preferentially cleaves the L-enantiomer, leaving D-HPAE intact . The reaction, conducted in aqueous media at ambient conditions, separates products via extraction: L-HPA remains in the aqueous phase, while D-HPAE is recovered organically . This method achieves >99% enantiomeric excess (ee) for L-HPA but requires racemization of the residual D-enantiomer to maintain stoichiometric efficiency .
Acetylation and Racemization
DE19546533A1 outlines a dynamic kinetic resolution (DKR) process. Racemic DL-HPA is acetylated to N-acetyl-DL-HPA, after which an acylase selectively deacetylates the L-enantiomer. The remaining N-acetyl-D-HPA is racemized using strong acids (e.g., HCl) or bases (e.g., NaOH) at elevated temperatures, regenerating the racemic mixture for recycling . This approach minimizes waste but demands robust enzyme stability and efficient racemization catalysts .
Comparative Analysis of Synthetic Routes
Chemical methods dominate industrial production due to lower enzyme costs and straightforward scalability. However, enzymatic routes gain traction for enantiopure demands, necessitating post-synthesis racemization for DL-HPA .
Industrial Production Considerations
Substrate Concentration and Cost
High substrate concentrations (e.g., 2 M OPBA in enzymatic reactions ) reduce volumetric costs but risk enzyme inhibition. Chemical methods tolerate higher loads, though reagent costs (e.g., cyanide) necessitate waste mitigation .
Crystallization and Purity
DL-HPA’s low solubility in aqueous buffers facilitates crystallization. Adjusting pH to the isoelectric point (pI ≈ 5.8) precipitates the compound, achieving >95% purity after recrystallization . In contrast, enzymatic resolutions require chiral columns or selective extractions, adding operational complexity .
Properties
IUPAC Name |
2-amino-4-phenylbutanoic acid | |
---|---|---|
Source | PubChem | |
URL | https://pubchem.ncbi.nlm.nih.gov | |
Description | Data deposited in or computed by PubChem | |
InChI |
InChI=1S/C10H13NO2/c11-9(10(12)13)7-6-8-4-2-1-3-5-8/h1-5,9H,6-7,11H2,(H,12,13) | |
Source | PubChem | |
URL | https://pubchem.ncbi.nlm.nih.gov | |
Description | Data deposited in or computed by PubChem | |
InChI Key |
JTTHKOPSMAVJFE-UHFFFAOYSA-N | |
Source | PubChem | |
URL | https://pubchem.ncbi.nlm.nih.gov | |
Description | Data deposited in or computed by PubChem | |
Canonical SMILES |
C1=CC=C(C=C1)CCC(C(=O)O)N | |
Source | PubChem | |
URL | https://pubchem.ncbi.nlm.nih.gov | |
Description | Data deposited in or computed by PubChem | |
Molecular Formula |
C10H13NO2 | |
Source | PubChem | |
URL | https://pubchem.ncbi.nlm.nih.gov | |
Description | Data deposited in or computed by PubChem | |
DSSTOX Substance ID |
DTXSID80874100 | |
Record name | HOMOPHENYLALANINE | |
Source | EPA DSSTox | |
URL | https://comptox.epa.gov/dashboard/DTXSID80874100 | |
Description | DSSTox provides a high quality public chemistry resource for supporting improved predictive toxicology. | |
Molecular Weight |
179.22 g/mol | |
Source | PubChem | |
URL | https://pubchem.ncbi.nlm.nih.gov | |
Description | Data deposited in or computed by PubChem | |
CAS No. |
1012-05-1 | |
Record name | 2-Amino-4-phenylbutyric acid | |
Source | ChemIDplus | |
URL | https://pubchem.ncbi.nlm.nih.gov/substance/?source=chemidplus&sourceid=0001012051 | |
Description | ChemIDplus is a free, web search system that provides access to the structure and nomenclature authority files used for the identification of chemical substances cited in National Library of Medicine (NLM) databases, including the TOXNET system. | |
Record name | HOMOPHENYLALANINE, DL- | |
Source | FDA Global Substance Registration System (GSRS) | |
URL | https://gsrs.ncats.nih.gov/ginas/app/beta/substances/NTL1IB8F3L | |
Description | The FDA Global Substance Registration System (GSRS) enables the efficient and accurate exchange of information on what substances are in regulated products. Instead of relying on names, which vary across regulatory domains, countries, and regions, the GSRS knowledge base makes it possible for substances to be defined by standardized, scientific descriptions. | |
Explanation | Unless otherwise noted, the contents of the FDA website (www.fda.gov), both text and graphics, are not copyrighted. They are in the public domain and may be republished, reprinted and otherwise used freely by anyone without the need to obtain permission from FDA. Credit to the U.S. Food and Drug Administration as the source is appreciated but not required. | |
Retrosynthesis Analysis
AI-Powered Synthesis Planning: Our tool employs the Template_relevance Pistachio, Template_relevance Bkms_metabolic, Template_relevance Pistachio_ringbreaker, Template_relevance Reaxys, Template_relevance Reaxys_biocatalysis model, leveraging a vast database of chemical reactions to predict feasible synthetic routes.
One-Step Synthesis Focus: Specifically designed for one-step synthesis, it provides concise and direct routes for your target compounds, streamlining the synthesis process.
Accurate Predictions: Utilizing the extensive PISTACHIO, BKMS_METABOLIC, PISTACHIO_RINGBREAKER, REAXYS, REAXYS_BIOCATALYSIS database, our tool offers high-accuracy predictions, reflecting the latest in chemical research and data.
Strategy Settings
Precursor scoring | Relevance Heuristic |
---|---|
Min. plausibility | 0.01 |
Model | Template_relevance |
Template Set | Pistachio/Bkms_metabolic/Pistachio_ringbreaker/Reaxys/Reaxys_biocatalysis |
Top-N result to add to graph | 6 |
Feasible Synthetic Routes
Disclaimer and Information on In-Vitro Research Products
Please be aware that all articles and product information presented on BenchChem are intended solely for informational purposes. The products available for purchase on BenchChem are specifically designed for in-vitro studies, which are conducted outside of living organisms. In-vitro studies, derived from the Latin term "in glass," involve experiments performed in controlled laboratory settings using cells or tissues. It is important to note that these products are not categorized as medicines or drugs, and they have not received approval from the FDA for the prevention, treatment, or cure of any medical condition, ailment, or disease. We must emphasize that any form of bodily introduction of these products into humans or animals is strictly prohibited by law. It is essential to adhere to these guidelines to ensure compliance with legal and ethical standards in research and experimentation.