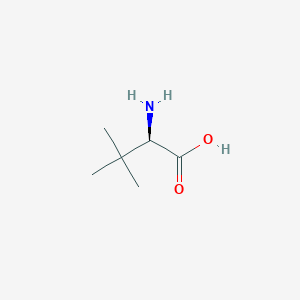
D-tert-Leucine
Overview
Description
D-tert-Leucine, also known as ®-2-amino-3,3-dimethylbutanoic acid, is a chiral, non-natural amino acid. It is characterized by its unique structure, which includes a tertiary butyl group attached to the alpha carbon. This compound is a white crystalline powder that is slightly soluble in water and more soluble in organic solvents like ethanol and ether .
Synthetic Routes and Reaction Conditions:
Chemical Synthesis: One common method involves the condensation of glyoxylate with chiral tert-butyl sulfinamide to form a Schiff base. This intermediate then reacts with tert-butyl Grignard reagent in the presence of a catalyst at low temperatures.
Biocatalysis: Another method utilizes a biocatalyst derived from Symbiobacterium thermophilum.
Industrial Production Methods:
- Industrial production often employs the biocatalysis method due to its high yield, purity, and potential for process scaling. The use of engineered enzymes and coenzyme recycling systems enhances the efficiency and sustainability of the production process .
Types of Reactions:
Oxidation: this compound can undergo oxidation reactions, typically involving reagents like hydrogen peroxide or potassium permanganate.
Reduction: Reduction reactions can be performed using reducing agents such as sodium borohydride or lithium aluminum hydride.
Substitution: Nucleophilic substitution reactions can occur, where the amino group is replaced by other functional groups using reagents like alkyl halides.
Common Reagents and Conditions:
Oxidation: Hydrogen peroxide in the presence of a catalyst.
Reduction: Sodium borohydride in methanol or lithium aluminum hydride in ether.
Substitution: Alkyl halides in the presence of a base like sodium hydroxide.
Major Products:
Oxidation: Products include carboxylic acids and ketones.
Reduction: Products include primary amines and alcohols.
Substitution: Products vary depending on the substituent introduced, such as alkylated amines.
Mechanism of Action
Target of Action
D-tert-Leucine, also known as ®-2-Amino-3,3-dimethylbutyric acid , is a chiral molecule that interacts with various targets in the bodyLeucine, a closely related amino acid, is known to interact with the l-type amino acid transporter (lat1) .
Mode of Action
It’s known that leucine and its derivatives can be taken up by cells via various transporters, including lat1 . The acetylation of leucine switches its uptake into cells from LAT1 to organic anion transporters (OAT1 and OAT3) and the monocarboxylate transporter type 1 (MCT1)
Biochemical Pathways
Leucine and its derivatives are known to be involved in various metabolic processes inside cells, such as mtor signaling . The low δ2H values of leucine and valine, as well as the consistent 2H-depletion of valine relative to leucine in glucose-grown organisms, may be attributed in part to low pyruvate δ2H values .
Pharmacokinetics
It’s known that the acetylation of leucine can enhance its pharmacokinetic properties . The kinetics of MCT1 (lower affinity compared to LAT1) and the ubiquitous tissue expression of MCT1 make it well suited for uptake and distribution of N-acetyl-L-leucine
Result of Action
It’s known that leucine and its derivatives can bypass lat1, the rate-limiting step in activation of leucine-mediated signaling and metabolic processes inside cells
Action Environment
It’s known that the enantiomers of n-acetyl-leucine show unexpected and large differences in pharmacokinetics, suggesting the involvement of differential binding sites provided by enzymes and transporters
Biochemical Analysis
Biochemical Properties
D-tert-Leucine is involved in various biochemical reactions. It interacts with a variety of enzymes, proteins, and other biomolecules. For instance, this compound is the target substrate for the D-amidase enzyme from Variovorax paradoxus . The nature of these interactions is primarily enzymatic, where this compound serves as a substrate for the enzyme.
Molecular Mechanism
At the molecular level, this compound exerts its effects through binding interactions with biomolecules, enzyme inhibition or activation, and changes in gene expression. For example, it acts as a substrate for the D-amidase enzyme, thereby influencing the enzyme’s activity .
Scientific Research Applications
D-tert-Leucine has a wide range of applications in scientific research:
Comparison with Similar Compounds
L-tert-Leucine: The L-enantiomer of tert-Leucine, used in similar applications but with different stereochemical properties.
Phenylalanine: An aromatic amino acid with distinct chemical behavior and applications.
Uniqueness:
- D-tert-Leucine’s unique tertiary butyl group provides steric hindrance, enhancing its stability and making it a valuable chiral template in asymmetric synthesis . Its non-natural configuration also allows for selective interactions in biological systems, distinguishing it from naturally occurring amino acids .
Properties
IUPAC Name |
(2R)-2-amino-3,3-dimethylbutanoic acid | |
---|---|---|
Source | PubChem | |
URL | https://pubchem.ncbi.nlm.nih.gov | |
Description | Data deposited in or computed by PubChem | |
InChI |
InChI=1S/C6H13NO2/c1-6(2,3)4(7)5(8)9/h4H,7H2,1-3H3,(H,8,9)/t4-/m0/s1 | |
Source | PubChem | |
URL | https://pubchem.ncbi.nlm.nih.gov | |
Description | Data deposited in or computed by PubChem | |
InChI Key |
NPDBDJFLKKQMCM-BYPYZUCNSA-N | |
Source | PubChem | |
URL | https://pubchem.ncbi.nlm.nih.gov | |
Description | Data deposited in or computed by PubChem | |
Canonical SMILES |
CC(C)(C)C(C(=O)O)N | |
Source | PubChem | |
URL | https://pubchem.ncbi.nlm.nih.gov | |
Description | Data deposited in or computed by PubChem | |
Isomeric SMILES |
CC(C)(C)[C@H](C(=O)O)N | |
Source | PubChem | |
URL | https://pubchem.ncbi.nlm.nih.gov | |
Description | Data deposited in or computed by PubChem | |
Molecular Formula |
C6H13NO2 | |
Source | PubChem | |
URL | https://pubchem.ncbi.nlm.nih.gov | |
Description | Data deposited in or computed by PubChem | |
DSSTOX Substance ID |
DTXSID30426193 | |
Record name | D-tert-Leucine | |
Source | EPA DSSTox | |
URL | https://comptox.epa.gov/dashboard/DTXSID30426193 | |
Description | DSSTox provides a high quality public chemistry resource for supporting improved predictive toxicology. | |
Molecular Weight |
131.17 g/mol | |
Source | PubChem | |
URL | https://pubchem.ncbi.nlm.nih.gov | |
Description | Data deposited in or computed by PubChem | |
CAS No. |
26782-71-8 | |
Record name | D-tert-Leucine | |
Source | CAS Common Chemistry | |
URL | https://commonchemistry.cas.org/detail?cas_rn=26782-71-8 | |
Description | CAS Common Chemistry is an open community resource for accessing chemical information. Nearly 500,000 chemical substances from CAS REGISTRY cover areas of community interest, including common and frequently regulated chemicals, and those relevant to high school and undergraduate chemistry classes. This chemical information, curated by our expert scientists, is provided in alignment with our mission as a division of the American Chemical Society. | |
Explanation | The data from CAS Common Chemistry is provided under a CC-BY-NC 4.0 license, unless otherwise stated. | |
Record name | 3-Methyl-D-valine | |
Source | ChemIDplus | |
URL | https://pubchem.ncbi.nlm.nih.gov/substance/?source=chemidplus&sourceid=0026782718 | |
Description | ChemIDplus is a free, web search system that provides access to the structure and nomenclature authority files used for the identification of chemical substances cited in National Library of Medicine (NLM) databases, including the TOXNET system. | |
Record name | D-tert-Leucine | |
Source | EPA DSSTox | |
URL | https://comptox.epa.gov/dashboard/DTXSID30426193 | |
Description | DSSTox provides a high quality public chemistry resource for supporting improved predictive toxicology. | |
Record name | 3-METHYL-D-VALINE | |
Source | FDA Global Substance Registration System (GSRS) | |
URL | https://gsrs.ncats.nih.gov/ginas/app/beta/substances/P3L3QZA16M | |
Description | The FDA Global Substance Registration System (GSRS) enables the efficient and accurate exchange of information on what substances are in regulated products. Instead of relying on names, which vary across regulatory domains, countries, and regions, the GSRS knowledge base makes it possible for substances to be defined by standardized, scientific descriptions. | |
Explanation | Unless otherwise noted, the contents of the FDA website (www.fda.gov), both text and graphics, are not copyrighted. They are in the public domain and may be republished, reprinted and otherwise used freely by anyone without the need to obtain permission from FDA. Credit to the U.S. Food and Drug Administration as the source is appreciated but not required. | |
Synthesis routes and methods I
Procedure details
Synthesis routes and methods II
Procedure details
Retrosynthesis Analysis
AI-Powered Synthesis Planning: Our tool employs the Template_relevance Pistachio, Template_relevance Bkms_metabolic, Template_relevance Pistachio_ringbreaker, Template_relevance Reaxys, Template_relevance Reaxys_biocatalysis model, leveraging a vast database of chemical reactions to predict feasible synthetic routes.
One-Step Synthesis Focus: Specifically designed for one-step synthesis, it provides concise and direct routes for your target compounds, streamlining the synthesis process.
Accurate Predictions: Utilizing the extensive PISTACHIO, BKMS_METABOLIC, PISTACHIO_RINGBREAKER, REAXYS, REAXYS_BIOCATALYSIS database, our tool offers high-accuracy predictions, reflecting the latest in chemical research and data.
Strategy Settings
Precursor scoring | Relevance Heuristic |
---|---|
Min. plausibility | 0.01 |
Model | Template_relevance |
Template Set | Pistachio/Bkms_metabolic/Pistachio_ringbreaker/Reaxys/Reaxys_biocatalysis |
Top-N result to add to graph | 6 |
Feasible Synthetic Routes
Disclaimer and Information on In-Vitro Research Products
Please be aware that all articles and product information presented on BenchChem are intended solely for informational purposes. The products available for purchase on BenchChem are specifically designed for in-vitro studies, which are conducted outside of living organisms. In-vitro studies, derived from the Latin term "in glass," involve experiments performed in controlled laboratory settings using cells or tissues. It is important to note that these products are not categorized as medicines or drugs, and they have not received approval from the FDA for the prevention, treatment, or cure of any medical condition, ailment, or disease. We must emphasize that any form of bodily introduction of these products into humans or animals is strictly prohibited by law. It is essential to adhere to these guidelines to ensure compliance with legal and ethical standards in research and experimentation.