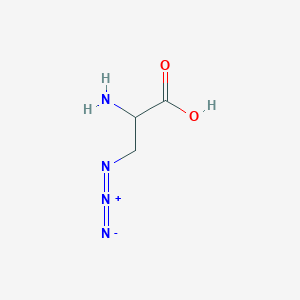
D-Alanine, 3-azido-
Overview
Description
D-Alanine, 3-azido-: is an azido-modified derivative of D-alanine, a naturally occurring amino acid. This compound is characterized by the presence of an azide group (-N₃) attached to the alanine molecule. The azide group imparts unique chemical properties to the compound, making it useful in various chemical and biological applications, particularly in the field of click chemistry.
Preparation Methods
Synthetic Routes and Reaction Conditions:
Starting Material: The synthesis of D-Alanine, 3-azido- typically begins with D-alanine.
Azidation Reaction: The azide group is introduced through a nucleophilic substitution reaction. D-alanine is reacted with sodium azide (NaN₃) in the presence of a suitable solvent, such as dimethyl sulfoxide (DMSO), under controlled temperature conditions.
Purification: The product is purified using techniques such as recrystallization or chromatography to obtain pure D-Alanine, 3-azido-.
Industrial Production Methods: While specific industrial production methods for D-Alanine, 3-azido- are not widely documented, the general approach involves scaling up the laboratory synthesis process. This includes optimizing reaction conditions, using industrial-grade reagents, and employing large-scale purification techniques to ensure high yield and purity.
Chemical Reactions Analysis
Types of Reactions:
Click Chemistry Reactions: D-Alanine, 3-azido- is prominently used in click chemistry, particularly in copper-catalyzed azide-alkyne cycloaddition (CuAAC) reactions. This reaction involves the azide group reacting with an alkyne group to form a stable triazole ring.
Ring Strain-Promoted Alkyne-Azide Cycloaddition (SPAAC): This reaction occurs without the need for a copper catalyst and involves the azide group reacting with strained alkynes such as dibenzocyclooctyne (DBCO) or bicyclononyne (BCN).
Common Reagents and Conditions:
Copper-Catalyzed Reactions: Copper sulfate (CuSO₄) and sodium ascorbate are commonly used as catalysts in CuAAC reactions.
Strained Alkynes: DBCO and BCN are used in SPAAC reactions.
Major Products:
Triazole Derivatives: The primary products of click chemistry reactions involving D-Alanine, 3-azido- are triazole derivatives, which are stable and have diverse applications in bioconjugation and material science.
Scientific Research Applications
Chemistry:
Click Chemistry Labeling: D-Alanine, 3-azido- is used as a labeling reagent in click chemistry to attach various functional groups to biomolecules, facilitating the study of molecular interactions and dynamics.
Biology:
Metabolic Labeling: This compound is incorporated into bacterial cell walls, enabling the study of bacterial growth and metabolism through fluorescence imaging techniques.
Medicine:
Drug Development: D-Alanine, 3-azido- is used in the development of novel drug delivery systems and therapeutic agents, particularly in targeting specific cellular pathways.
Industry:
Material Science: The compound is used in the synthesis of advanced materials with specific properties, such as enhanced stability and reactivity, for industrial applications.
Mechanism of Action
Molecular Targets and Pathways:
Azide Group Reactivity: The azide group in D-Alanine, 3-azido- is highly reactive and participates in cycloaddition reactions, forming stable triazole rings. This reactivity is harnessed in click chemistry to create bioconjugates and functionalized materials.
Incorporation into Biomolecules: In biological systems, D-Alanine, 3-azido- is incorporated into proteins and cell walls, allowing for precise labeling and tracking of biological processes.
Comparison with Similar Compounds
3-Azido-L-alanine: Similar to D-Alanine, 3-azido-, but with the L-configuration of alanine.
Azidohomoalanine: An azido-modified derivative of homoalanine, used in similar click chemistry applications.
Azidolysine: An azido-modified derivative of lysine, used for labeling and bioconjugation.
Uniqueness:
Stereochemistry: The D-configuration of D-Alanine, 3-azido- distinguishes it from its L-counterpart, affecting its incorporation into biological systems and its reactivity in stereospecific reactions.
Versatility: The azide group in D-Alanine, 3-azido- provides a versatile handle for various chemical modifications, making it a valuable tool in both research and industrial applications.
Properties
IUPAC Name |
2-amino-3-azidopropanoic acid | |
---|---|---|
Source | PubChem | |
URL | https://pubchem.ncbi.nlm.nih.gov | |
Description | Data deposited in or computed by PubChem | |
InChI |
InChI=1S/C3H6N4O2/c4-2(3(8)9)1-6-7-5/h2H,1,4H2,(H,8,9) | |
Source | PubChem | |
URL | https://pubchem.ncbi.nlm.nih.gov | |
Description | Data deposited in or computed by PubChem | |
InChI Key |
CIFCKCQAKQRJFC-UHFFFAOYSA-N | |
Source | PubChem | |
URL | https://pubchem.ncbi.nlm.nih.gov | |
Description | Data deposited in or computed by PubChem | |
Canonical SMILES |
C(C(C(=O)O)N)N=[N+]=[N-] | |
Source | PubChem | |
URL | https://pubchem.ncbi.nlm.nih.gov | |
Description | Data deposited in or computed by PubChem | |
Molecular Formula |
C3H6N4O2 | |
Source | PubChem | |
URL | https://pubchem.ncbi.nlm.nih.gov | |
Description | Data deposited in or computed by PubChem | |
DSSTOX Substance ID |
DTXSID601030553 | |
Record name | 3-Azidoalanine | |
Source | EPA DSSTox | |
URL | https://comptox.epa.gov/dashboard/DTXSID601030553 | |
Description | DSSTox provides a high quality public chemistry resource for supporting improved predictive toxicology. | |
Molecular Weight |
130.11 g/mol | |
Source | PubChem | |
URL | https://pubchem.ncbi.nlm.nih.gov | |
Description | Data deposited in or computed by PubChem | |
CAS No. |
88192-18-1, 105928-88-9, 108342-09-2 | |
Record name | Azidoalanine | |
Source | ChemIDplus | |
URL | https://pubchem.ncbi.nlm.nih.gov/substance/?source=chemidplus&sourceid=0088192181 | |
Description | ChemIDplus is a free, web search system that provides access to the structure and nomenclature authority files used for the identification of chemical substances cited in National Library of Medicine (NLM) databases, including the TOXNET system. | |
Record name | Alanine, 3-azido-, D- | |
Source | ChemIDplus | |
URL | https://pubchem.ncbi.nlm.nih.gov/substance/?source=chemidplus&sourceid=0105928889 | |
Description | ChemIDplus is a free, web search system that provides access to the structure and nomenclature authority files used for the identification of chemical substances cited in National Library of Medicine (NLM) databases, including the TOXNET system. | |
Record name | Alanine, 3-azido-, DL- | |
Source | ChemIDplus | |
URL | https://pubchem.ncbi.nlm.nih.gov/substance/?source=chemidplus&sourceid=0108342092 | |
Description | ChemIDplus is a free, web search system that provides access to the structure and nomenclature authority files used for the identification of chemical substances cited in National Library of Medicine (NLM) databases, including the TOXNET system. | |
Record name | 3-Azidoalanine | |
Source | EPA DSSTox | |
URL | https://comptox.epa.gov/dashboard/DTXSID601030553 | |
Description | DSSTox provides a high quality public chemistry resource for supporting improved predictive toxicology. | |
Synthesis routes and methods
Procedure details
Retrosynthesis Analysis
AI-Powered Synthesis Planning: Our tool employs the Template_relevance Pistachio, Template_relevance Bkms_metabolic, Template_relevance Pistachio_ringbreaker, Template_relevance Reaxys, Template_relevance Reaxys_biocatalysis model, leveraging a vast database of chemical reactions to predict feasible synthetic routes.
One-Step Synthesis Focus: Specifically designed for one-step synthesis, it provides concise and direct routes for your target compounds, streamlining the synthesis process.
Accurate Predictions: Utilizing the extensive PISTACHIO, BKMS_METABOLIC, PISTACHIO_RINGBREAKER, REAXYS, REAXYS_BIOCATALYSIS database, our tool offers high-accuracy predictions, reflecting the latest in chemical research and data.
Strategy Settings
Precursor scoring | Relevance Heuristic |
---|---|
Min. plausibility | 0.01 |
Model | Template_relevance |
Template Set | Pistachio/Bkms_metabolic/Pistachio_ringbreaker/Reaxys/Reaxys_biocatalysis |
Top-N result to add to graph | 6 |
Feasible Synthetic Routes
Disclaimer and Information on In-Vitro Research Products
Please be aware that all articles and product information presented on BenchChem are intended solely for informational purposes. The products available for purchase on BenchChem are specifically designed for in-vitro studies, which are conducted outside of living organisms. In-vitro studies, derived from the Latin term "in glass," involve experiments performed in controlled laboratory settings using cells or tissues. It is important to note that these products are not categorized as medicines or drugs, and they have not received approval from the FDA for the prevention, treatment, or cure of any medical condition, ailment, or disease. We must emphasize that any form of bodily introduction of these products into humans or animals is strictly prohibited by law. It is essential to adhere to these guidelines to ensure compliance with legal and ethical standards in research and experimentation.