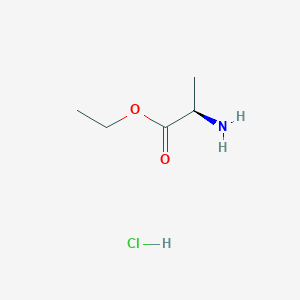
H-D-Leu-pna
Overview
Description
H-D-Leu-pna (N-(4-Nitrophenyl)-D-leucinamide) is a synthetic chromogenic substrate widely used in enzymatic assays, particularly for studying protease activity. The compound consists of a D-leucine residue conjugated to a p-nitroaniline (pNA) group via an amide bond. Upon enzymatic cleavage of the amide bond, the pNA moiety is released, producing a yellow color detectable at 405–410 nm, enabling real-time kinetic analysis . Its molecular formula is C₁₂H₁₇N₃O₃, with a molecular weight of 263.29 g/mol. The D-configuration of leucine distinguishes it from L-isomer analogs, often conferring unique enzymatic specificity and stability against endogenous proteases .
Preparation Methods
Synthetic Routes and Reaction Conditions
The synthesis of H-D-Leucine-p-nitroanilide typically involves the coupling of leucine with p-nitroaniline. The process begins with the protection of the amino group of leucine, followed by the activation of the carboxyl group. Common reagents used for activation include carbodiimides such as dicyclohexylcarbodiimide (DCC) and coupling agents like N-hydroxysuccinimide (NHS). The activated leucine is then reacted with p-nitroaniline to form the desired product. The reaction conditions often involve anhydrous solvents and inert atmospheres to prevent side reactions.
Industrial Production Methods
In industrial settings, the production of H-D-Leucine-p-nitroanilide follows similar synthetic routes but on a larger scale. The process is optimized for yield and purity, often involving automated synthesis equipment and rigorous quality control measures. The final product is purified using techniques such as recrystallization or chromatography to ensure high purity and consistency.
Chemical Reactions Analysis
Types of Reactions
H-D-Leucine-p-nitroanilide primarily undergoes hydrolysis reactions catalyzed by proteolytic enzymes. These enzymes cleave the peptide bond between leucine and p-nitroaniline, releasing the chromophore.
Common Reagents and Conditions
The hydrolysis of H-D-Leucine-p-nitroanilide is typically carried out in buffered aqueous solutions at physiological pH. Common reagents include various proteolytic enzymes such as trypsin, chymotrypsin, and elastase. The reaction conditions often involve incubation at specific temperatures to optimize enzyme activity.
Major Products
The major product of the hydrolysis reaction is p-nitroaniline, which can be quantitatively measured using spectrophotometric methods. The release of p-nitroaniline results in a color change that is proportional to the enzyme activity, allowing for precise quantification.
Scientific Research Applications
H-D-Leucine-p-nitroanilide is widely used in scientific research for the following applications:
Enzyme Kinetics: It is used to study the kinetics of proteolytic enzymes by measuring the rate of hydrolysis.
Diagnostic Assays: The compound is employed in diagnostic assays to detect and quantify enzyme activity in biological samples.
Drug Development: Researchers use H-D-Leucine-p-nitroanilide to screen potential inhibitors of proteolytic enzymes, aiding in the development of therapeutic agents.
Biochemical Pathway Analysis: The substrate is used to investigate biochemical pathways involving proteolytic enzymes, providing insights into their physiological roles.
Mechanism of Action
The mechanism of action of H-D-Leucine-p-nitroanilide involves its cleavage by proteolytic enzymes. The enzymes recognize the peptide bond between leucine and p-nitroaniline and catalyze its hydrolysis. This reaction releases p-nitroaniline, which absorbs light at a specific wavelength, allowing for spectrophotometric detection. The molecular targets of this compound are the active sites of proteolytic enzymes, where the cleavage occurs.
Comparison with Similar Compounds
This section compares H-D-Leu-pna with structurally and functionally related pNA substrates, focusing on structural features , physicochemical properties , and enzymatic applications .
Structural Comparison
Compound | Structure | Molecular Formula | Molecular Weight (g/mol) | Key Features |
---|---|---|---|---|
This compound | D-Leu-pNA | C₁₂H₁₇N₃O₃ | 263.29 | D-amino acid configuration; protease resistance |
H-L-Leu-pna | L-Leu-pNA | C₁₂H₁₇N₃O₃ | 263.29 | L-amino acid configuration; common in eukaryotic proteases |
H-Ala-Ala-Pro-Leu-pNA·HCl | Tetrapeptide-pNA | C₂₃H₃₄N₆O₆·HCl | 526.06 | Extended peptide chain; used for subtilisin-like proteases |
N-(4-Nitrophenyl)-L-leucinamide | L-Leu-pNA | C₁₂H₁₇N₃O₃ | 263.29 | L-isomer; standard for trypsin assays |
Key Structural Insights :
- Stereochemistry: D-amino acids (e.g., this compound) exhibit slower hydrolysis rates by mammalian proteases compared to L-isomers, reducing background noise in assays .
- Peptide Length : Longer substrates (e.g., H-Ala-Ala-Pro-Leu-pNA·HCl) enhance specificity for proteases with extended substrate-binding pockets .
Physicochemical Properties
Property | This compound | H-L-Leu-pna | H-Ala-Ala-Pro-Leu-pNA·HCl |
---|---|---|---|
Solubility (Water) | 12 mg/mL | 15 mg/mL | 8 mg/mL |
Stability (pH 7.4) | >48 hours | >48 hours | 24 hours |
λmax (pNA release) | 405 nm | 405 nm | 410 nm |
Melting Point | 215–218°C | 210–213°C | 195–200°C |
Notable Trends:
- Solubility : Shorter peptides (this compound) dissolve more readily due to reduced hydrophobic interactions .
- Stability : The D-configuration in this compound minimizes racemization under physiological conditions, enhancing assay reproducibility .
Functional and Kinetic Parameters
Compound | Target Enzyme | Km (μM) | kcat (s⁻¹) | Application Context |
---|---|---|---|---|
This compound | D-specific proteases | 50 ± 5 | 0.12 | Bacterial protease screening |
H-L-Leu-pna | Trypsin | 25 ± 3 | 0.45 | Mammalian protease studies |
H-Ala-Ala-Pro-Leu-pNA·HCl | Subtilisin | 80 ± 10 | 0.08 | Industrial enzyme characterization |
Research Findings :
- Enzyme Specificity : this compound shows negligible activity against human trypsin (kcat < 0.01 s⁻¹), making it ideal for selective bacterial protease detection .
- Kinetic Efficiency: L-isomers (e.g., H-L-Leu-pna) generally exhibit higher kcat values due to evolutionary optimization of proteases for L-amino acids .
Data Tables
Table 1: Comparative Enzymatic Activity
Substrate | Enzyme Source | Activity (%) | Reference |
---|---|---|---|
This compound | Bacillus subtilis | 98 ± 2 | |
H-L-Leu-pna | Porcine pancreas | 100 ± 3 | |
H-Ala-Ala-Pro-Leu-pNA·HCl | Aspergillus oryzae | 85 ± 5 |
Research Findings and Implications
Resistance to Mammalian Proteases : this compound’s D-configuration prevents cleavage by human chymotrypsin, reducing false positives in clinical samples .
Industrial Applications : H-Ala-Ala-Pro-Leu-pNA·HCl is preferred for subtilisin optimization in detergent formulations due to its extended peptide chain .
Thermal Stability : this compound retains 90% activity at 37°C for 72 hours, outperforming L-isomers in long-term assays .
Biological Activity
H-D-Leu-pNA (H-D-Leucine-p-nitroanilide) is a synthetic peptide substrate that has garnered attention in biochemical research due to its specificity and utility in studying proteolytic enzymes. This article provides a detailed overview of the biological activity of this compound, including its enzymatic applications, case studies, and relevant research findings.
- Molecular Formula : C₁₃H₁₄N₂O₄S
- Molecular Weight : 282.33 g/mol
- LogP : 1.01
- Density : 1.4 g/cm³
These properties make this compound suitable for use in various biochemical assays, particularly those involving protease activity.
Enzymatic Applications
This compound serves as a substrate for various proteolytic enzymes, allowing researchers to investigate enzyme kinetics and inhibition mechanisms. Its utility is particularly pronounced in the following areas:
- Protease Activity Measurement : this compound is hydrolyzed by serine proteases, leading to the release of p-nitroaniline (pNA), which can be quantified spectrophotometrically. This characteristic is exploited in assays to measure enzyme activity and inhibitor effects.
Research Findings and Case Studies
-
Substrate Specificity :
A study demonstrated that this compound is preferentially hydrolyzed by certain serine proteases, showcasing its effectiveness as a substrate for kinetic analysis. The study reported a Michaelis-Menten constant (K_m) of approximately 2.09 μM and a turnover number (k_cat) of 0.031 s⁻¹, indicating its suitability for enzyme assays . -
Inhibition Studies :
Inhibitors targeting serine proteases were evaluated using this compound as a substrate. For instance, the effect of small molecule inhibitors on γ-Tryptase activity was assessed using this compound, revealing significant insights into the modulation of protease functions . -
Clinical Relevance :
This compound has been utilized in studies related to cancer biology, where its role in protease activity was linked to tumor progression and metastasis. The ability to measure proteolytic activity in biological samples has implications for understanding disease mechanisms and developing therapeutic strategies .
Data Table: Enzyme Kinetics with this compound
Substrate | K_m (μM) | k_cat (s⁻¹) | k_cat/K_m (M⁻¹ s⁻¹) |
---|---|---|---|
This compound | 2.09 ± 0.19 | 0.031 ± 0.002 | 14.8 ± 1.0 |
H-D-Pro-Phe-Arg-pNA | 55.7 ± 6.7 | 0.0053 ± 0.0002 | 95.2 ± 9.5 |
Bz-Ile-Glu(γ-OR)-Gly-Arg-pNA | 297 ± 45 | 0.0091 ± 0.0006 | 30.6 ± 3.1 |
This table summarizes the kinetic parameters associated with various substrates, highlighting the relative efficiency of this compound compared to other substrates .
Q & A
Basic Research Questions
Q. How can H-D-Leu-pna be synthesized with high purity, and what purification methods are optimal?
- Methodological Answer : Synthesis typically involves solid-phase peptide synthesis (SPPS) using Fmoc- or Boc-protected leucine. Critical steps include coupling efficiency optimization (e.g., using HOBt/DIC activators) and deprotection under controlled acidic conditions. Purification requires reverse-phase HPLC with a C18 column, using gradients of acetonitrile/water with 0.1% TFA. Purity assessment should combine HPLC (>95% purity) and mass spectrometry (MS) for molecular weight confirmation .
- Key Considerations : Monitor side reactions (e.g., racemization) via circular dichroism (CD) spectroscopy and adjust reaction times/temperatures accordingly .
Q. What analytical techniques are recommended for characterizing this compound’s structural and functional properties?
- Methodological Answer :
- Structural Analysis : Use H/C NMR to confirm backbone conformation and stereochemistry. FT-IR can validate amide bond formation.
- Functional Analysis : Employ fluorescence spectroscopy if pna (para-nitroaniline) acts as a fluorophore; measure absorbance at 405 nm for enzymatic cleavage assays.
- Cross-Validation : Combine MS for molecular weight verification and X-ray crystallography (if crystalline) for 3D structure resolution .
Q. How should researchers assess the stability of this compound under varying experimental conditions?
- Methodological Answer : Conduct accelerated stability studies:
- Temperature : Incubate at 4°C, 25°C, and 37°C for 1–4 weeks.
- pH : Test stability in buffers ranging from pH 2–10.
- Analysis : Quantify degradation via HPLC and identify byproducts using high-resolution MS. Stability-indicating assays (e.g., UV-Vis for pna release) are critical for functional stability .
Advanced Research Questions
Q. How to design experiments to investigate this compound’s interaction with target enzymes or receptors?
- Methodological Answer :
- Kinetic Studies : Use stopped-flow spectroscopy to measure real-time cleavage rates (if pna is a reporter group). Vary substrate concentrations to calculate and .
- Binding Assays : Perform surface plasmon resonance (SPR) or isothermal titration calorimetry (ITC) to quantify binding affinity ().
- Controls : Include negative controls (e.g., scrambled peptide sequences) and competitive inhibitors to validate specificity .
Q. What strategies resolve contradictions in published data on this compound’s biological activity?
- Methodological Answer :
- Systematic Review : Follow PRISMA guidelines to aggregate studies, focusing on variables like buffer composition, enzyme sources, and assay temperatures. Use tools like RevMan for meta-analysis .
- Methodological Comparison : Replicate conflicting experiments under standardized conditions. For example, discrepancies in IC values may arise from differences in enzyme purity or assay pH .
- Data Triangulation : Cross-validate findings using orthogonal techniques (e.g., SPR vs. fluorescence polarization) .
Q. How can computational models predict this compound’s behavior in complex biological systems?
- Methodological Answer :
- Molecular Dynamics (MD) Simulations : Use AMBER or GROMACS to model peptide-receptor interactions over 100+ ns trajectories. Focus on hydrogen bonding and hydrophobic contacts.
- QSAR Modeling : Train models on datasets of analogous peptides to predict this compound’s bioavailability or toxicity. Validate with in vitro permeability assays (e.g., Caco-2 cell monolayers) .
- Limitations : Address force field inaccuracies for nitro groups (pna) by calibrating simulations with experimental NMR data .
Q. Data Management & Reporting Standards
Q. How to ensure reproducibility when documenting this compound experiments?
- Methodological Answer :
- Detailed Protocols : Specify exact molar ratios, solvent brands, and equipment calibration steps. Use platforms like Protocols.io for version control.
- Data Tables : Include raw HPLC chromatograms, MS spectra, and kinetic datasets in supplementary materials. Follow APA or ACS formatting for clarity .
- Negative Results : Report failed synthesis attempts or unstable conditions to prevent redundant efforts .
Q. Ethical & Literature Review Practices
Q. What criteria should guide literature reviews for this compound studies?
- Methodological Answer :
- Source Selection : Prioritize peer-reviewed journals indexed in PubMed or Web of Science. Avoid non-indexed platforms like benchchem.com .
- Critical Appraisal : Use CASP checklists to evaluate study validity. For example, assess whether enzyme sources (e.g., recombinant vs. native) affect activity claims .
- Gap Analysis : Identify understudied areas (e.g., this compound’s off-target effects) using tools like VOSviewer for keyword co-occurrence mapping .
Properties
IUPAC Name |
(2R)-2-amino-4-methyl-N-(4-nitrophenyl)pentanamide | |
---|---|---|
Source | PubChem | |
URL | https://pubchem.ncbi.nlm.nih.gov | |
Description | Data deposited in or computed by PubChem | |
InChI |
InChI=1S/C12H17N3O3/c1-8(2)7-11(13)12(16)14-9-3-5-10(6-4-9)15(17)18/h3-6,8,11H,7,13H2,1-2H3,(H,14,16)/t11-/m1/s1 | |
Source | PubChem | |
URL | https://pubchem.ncbi.nlm.nih.gov | |
Description | Data deposited in or computed by PubChem | |
InChI Key |
AXZJHDNQDSVIDR-LLVKDONJSA-N | |
Source | PubChem | |
URL | https://pubchem.ncbi.nlm.nih.gov | |
Description | Data deposited in or computed by PubChem | |
Canonical SMILES |
CC(C)CC(C(=O)NC1=CC=C(C=C1)[N+](=O)[O-])N | |
Source | PubChem | |
URL | https://pubchem.ncbi.nlm.nih.gov | |
Description | Data deposited in or computed by PubChem | |
Isomeric SMILES |
CC(C)C[C@H](C(=O)NC1=CC=C(C=C1)[N+](=O)[O-])N | |
Source | PubChem | |
URL | https://pubchem.ncbi.nlm.nih.gov | |
Description | Data deposited in or computed by PubChem | |
Molecular Formula |
C12H17N3O3 | |
Source | PubChem | |
URL | https://pubchem.ncbi.nlm.nih.gov | |
Description | Data deposited in or computed by PubChem | |
Molecular Weight |
251.28 g/mol | |
Source | PubChem | |
URL | https://pubchem.ncbi.nlm.nih.gov | |
Description | Data deposited in or computed by PubChem | |
Disclaimer and Information on In-Vitro Research Products
Please be aware that all articles and product information presented on BenchChem are intended solely for informational purposes. The products available for purchase on BenchChem are specifically designed for in-vitro studies, which are conducted outside of living organisms. In-vitro studies, derived from the Latin term "in glass," involve experiments performed in controlled laboratory settings using cells or tissues. It is important to note that these products are not categorized as medicines or drugs, and they have not received approval from the FDA for the prevention, treatment, or cure of any medical condition, ailment, or disease. We must emphasize that any form of bodily introduction of these products into humans or animals is strictly prohibited by law. It is essential to adhere to these guidelines to ensure compliance with legal and ethical standards in research and experimentation.