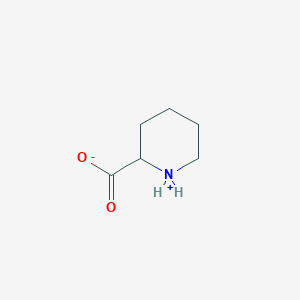
Pipecolic acid
Overview
Description
Pipecolic acid (2-piperidinecarboxylic acid) is a cyclic, non-proteinogenic amino acid with the molecular formula C₆H₁₁NO₂ and a molecular weight of 129.16 g/mol . It features a six-membered piperidine ring with a carboxylic acid functional group, distinguishing it from proteinogenic amino acids. Naturally occurring in plants, microbes, and animals, this compound serves as a precursor for bioactive compounds such as immunosuppressants (e.g., rapamycin, FK506) , anticancer agents (e.g., VX710) , and plant defense signaling molecules . Its biosynthesis primarily occurs via lysine metabolism, involving intermediates like Δ¹-piperideine-2-carboxylic acid (P2C) and Δ¹-piperideine-6-carboxylic acid (P6C) .
Mechanism of Action
Piperidine-2-carboxylic acid, also known as DL-Pipecolinic acid or Pipecolic acid, is an organic compound with the formula HNC5H9CO2H .
Target of Action
Piperidine-2-carboxylic acid’s primary target is the Gag-Pol polyprotein in HIV-2 . This protein plays a crucial role in the life cycle of the HIV-2 virus, making it a significant target for antiviral drugs .
Mode of Action
It is known to interact with its target, leading to changes that inhibit the function of the gag-pol polyprotein . This interaction disrupts the life cycle of the HIV-2 virus, thereby exerting its antiviral effects .
Biochemical Pathways
Piperidine-2-carboxylic acid is involved in various biochemical pathways. Its biosynthesis starts from lysine . It is also known to affect several crucial signaling pathways essential for the establishment of cancers such as STAT-3, NF-κB, PI3k/Aκt, JNK/p38-MAPK, TGF-ß/SMAD, Smac/DIABLO, p-IκB .
Result of Action
Piperidine-2-carboxylic acid has been observed to have therapeutic properties, including anticancer potential . It has been found to inhibit cell migration and help in cell cycle arrest, thereby inhibiting the survivability of cancer cells .
Biochemical Analysis
Biochemical Properties
Piperidine-2-carboxylic acid is an intermediate in the lysine degradation pathway . It interacts with enzymes such as lysine decarboxylase and copper amine oxidase in this pathway . It can be analyzed using tandem mass spectrometry and stable isotope dilution gas chromatography/mass spectrometry .
Cellular Effects
Piperidine-2-carboxylic acid has been observed to have effects on various types of cells. For instance, it has been associated with some forms of epilepsy, such as pyridoxine-dependent epilepsy . This suggests that piperidine-2-carboxylic acid may influence cell signaling pathways and gene expression related to neurological function .
Molecular Mechanism
The molecular mechanism of piperidine-2-carboxylic acid involves its interactions with biomolecules. For example, it is known to bind with enzymes in the lysine degradation pathway . This binding can lead to enzyme activation or inhibition, influencing the metabolic processes within the cell .
Metabolic Pathways
Piperidine-2-carboxylic acid is involved in the lysine degradation pathway . It interacts with enzymes such as lysine decarboxylase and copper amine oxidase in this pathway .
Biological Activity
Pipecolic acid (Pip), a cyclic non-protein amino acid derived from L-lysine, has garnered significant attention due to its diverse biological activities and potential applications in various fields, including medicine and agriculture. This article provides a comprehensive overview of the biological activity of this compound, highlighting its synthesis, mechanisms of action, and implications in health and disease.
1. Chemical Structure and Synthesis
This compound is characterized by a six-membered ring containing five carbon atoms and one nitrogen atom. Its structure allows it to participate in various biochemical pathways. The biosynthesis of this compound primarily occurs through the transamination of L-lysine, facilitated by specific enzymes such as ALD1 (a lysine-derived aminotransferase) that catalyze the conversion of L-lysine to this compound via intermediates like 1,2-dehydrothis compound (DP) .
2.1 Plant Immunity
Research has established that this compound plays a crucial role in plant systemic acquired resistance (SAR). It acts as a signaling molecule that enhances the plant's immune response against bacterial pathogens. Studies have shown that upon pathogen attack, this compound levels increase, which correlates with enhanced resistance to subsequent infections .
Table 1: Role of this compound in Plant Immunity
Study Reference | Organism | Observed Effect |
---|---|---|
Návarová et al., 2012 | Arabidopsis thaliana | Increased SAR upon pathogen inoculation |
Zeier, 2013 | Various plants | Enhanced resistance to bacterial infections |
2.2 Neurotransmitter Mimicry
This compound exhibits GABA-mimetic properties, acting as a neuromodulator in the central nervous system. It has been shown to influence neurotransmission by mimicking the action of gamma-aminobutyric acid (GABA), which is critical for inhibitory signaling in the brain. This property suggests potential therapeutic applications for conditions such as anxiety and epilepsy .
3. Antimicrobial Activity
The antimicrobial properties of this compound have been explored extensively, particularly in relation to its derivatives. For instance, studies indicate that certain this compound-containing compounds exhibit significant activity against various pathogens, including drug-resistant strains of bacteria and fungi .
Table 2: Antimicrobial Activity of this compound Derivatives
Compound | Target Organism | Activity Level |
---|---|---|
Tripropeptin Cpip | Staphylococcus aureus | Moderate |
Rapamycin | Candida albicans | High |
4.1 Pharmaceutical Applications
Recent advancements have highlighted the potential of this compound as a precursor for synthesizing novel pharmaceuticals. For example, derivatives of this compound have been incorporated into peptide structures to enhance their biological activity and stability .
4.2 Metabolic Disorders
This compound levels have been implicated in various metabolic disorders. Elevated levels are often associated with conditions such as hyperlysinemia and certain types of cancer, indicating its potential role as a biomarker for disease diagnosis .
5. Conclusion
The biological activity of this compound is multifaceted, impacting areas from plant immunity to human health. Its ability to act as a signaling molecule in plants and a GABA-mimetic agent in mammals underscores its importance across different biological systems. Ongoing research into its synthesis and applications continues to reveal new therapeutic potentials, making this compound a compound of significant interest in both pharmaceutical and agricultural sciences.
Scientific Research Applications
Plant Immunity and Systemic Acquired Resistance
Pipecolic acid plays a crucial role in enhancing systemic acquired resistance (SAR) in plants. Research has demonstrated that it acts as a signaling molecule that regulates immunity against bacterial pathogens.
- Mechanism of Action : this compound accumulates in plants during pathogen infection, promoting the production of reactive oxygen species (ROS) and nitric oxide (NO), which are essential for SAR. Studies indicate that the synthesis of this compound is dependent on salicylic acid and glycerol-3-phosphate, linking it to established immune pathways .
- Case Study : In Arabidopsis thaliana, localized application of this compound significantly reduced the growth of Pseudomonas syringae, demonstrating its potential as a biocontrol agent in agriculture. This effect was observed even in plants genetically modified to be deficient in this compound synthesis, suggesting its critical role in activating defense mechanisms .
Pharmaceutical Applications
This compound has shown promise in various pharmaceutical contexts, particularly in drug development and disease treatment.
- Anti-Cancer Potential : Recent studies have indicated that this compound derivatives may possess anti-cancer properties. It has been suggested that these compounds can enhance the efficacy of existing chemotherapeutic agents by modulating metabolic pathways involved in tumor growth .
- Neuroprotective Effects : Research has highlighted this compound's neuroprotective capabilities, particularly in models of neurodegenerative diseases. Its ability to modulate oxidative stress and inflammatory responses makes it a candidate for therapeutic interventions .
Biotechnological Applications
In biotechnology, this compound is utilized for its metabolic properties and as a precursor for synthesizing various compounds.
- Microbial Production : this compound can be produced using recombinant strains of Corynebacterium glutamicum, which expands the natural lysine biosynthetic pathway. This biotechnological method offers a sustainable approach to producing this compound at scale for industrial applications .
- Compatible Solute : In microbial physiology, this compound functions as a compatible solute that helps organisms cope with osmotic stress. This property is particularly valuable in developing stress-resistant microbial strains for agricultural or industrial use .
Clinical Implications
The clinical relevance of this compound is underscored by its association with certain metabolic disorders.
- Diagnostic Marker : Elevated levels of this compound have been observed in patients with peroxisomal disorders, making it a potential biomarker for diagnostic purposes. Its measurement could aid in the early detection and management of these conditions .
Data Summary Table
Application Area | Description | Key Findings |
---|---|---|
Plant Immunity | Enhances systemic acquired resistance | Reduces pathogen growth significantly |
Pharmaceutical Development | Potential anti-cancer and neuroprotective properties | Modulates metabolic pathways |
Biotechnological Production | Produced via recombinant strains; functions as a compatible solute | Sustainable production methods |
Clinical Diagnostics | Elevated levels indicate peroxisomal disorders | Potential biomarker for early diagnosis |
Q & A
Basic Research Questions
Q. What is the primary role of pipecolic acid (Pip) in plant systemic acquired resistance (SAR), and how is its accumulation measured experimentally?
this compound is a key signaling molecule in SAR, facilitating long-range defense priming against pathogens. Its accumulation can be quantified using high-performance liquid chromatography (HPLC) coupled with mass spectrometry (MS). For example, in barley, Pip levels were measured in HvALD1 CRISPR/Cas9 mutants after infection with Pseudomonas syringae to confirm the gene's role in Pip biosynthesis . Baseline Pip levels in mutants suggest alternative biosynthetic pathways or compensatory genes, requiring validation via knockout studies or enzymatic assays .
Q. What are the two primary biosynthetic routes of this compound in microorganisms, and how do they differ?
Microbial Pip biosynthesis occurs via two lysine-derived pathways:
- Route 1 : Loss of the α-amino group of lysine, forming D1-piperideine-2-carboxylic acid (P2C), which equilibrates with α-keto-ε-amino-caproic acid.
- Route 2 : Loss of the ε-nitrogen, yielding D1-piperideine-6-carboxylic acid (P6C), which equilibrates with α-aminoadipic-δ-semialdehyde. These routes are distinguished by the nitrogen retained in Pip and have been validated in microbes through isotopic labeling and enzyme characterization (e.g., aminotransferases) .
Q. How can researchers distinguish endogenous Pip functions from exogenous effects in plant immunity studies?
Methodologies include:
- Using genetic mutants (e.g., HvALD1 CRISPR mutants in barley) to disrupt endogenous Pip production while applying exogenous Pip to test rescue effects .
- Employing isotopic labeling (e.g., ¹⁵N-lysine) to trace Pip biosynthesis in wild-type vs. mutant plants .
- Profiling defense markers (e.g., PR genes) and volatile organic compounds (VOCs) like nonanal, which are Pip-dependent in SAR .
Advanced Research Questions
Q. What experimental strategies address contradictory data on baseline this compound levels in HvALD1 mutants?
In barley HvALD1 mutants, baseline Pip persists despite disrupted infection-induced accumulation, suggesting:
- Alternative pathways : Screen for redundant enzymes (e.g., lysine cyclodeaminases) via transcriptomics or proteomics.
- Compartmentalization : Localize Pip synthesis using tissue-specific promoters or subcellular fractionation.
- Cross-species validation : Compare Pip biosynthesis in Arabidopsis ald1 mutants (where baseline Pip is absent) to identify monocot-specific mechanisms .
Q. How can stereochemical purity of this compound derivatives be ensured during synthetic preparation for pharmacological studies?
- Chiral resolution : Use enantioselective chromatography (e.g., chiral HPLC columns) or enzymatic resolution with acylases.
- Asymmetric synthesis : Employ L- or D-specific enzymes (e.g., dehydrogenases) in microbial systems, as demonstrated in the stereoselective synthesis of (S)-pipecolic acid amides for antimicrobial agents .
- Crystallography : Verify purity via X-ray diffraction of derivatives, as in the characterization of (S)-pipecolic acid intermediates .
Q. What molecular mechanisms link this compound to plant-to-plant communication, and how can these be experimentally validated?
Pip primes neighboring plants via airborne VOCs like nonanal. Methodologies include:
- Volatile profiling : Use gas chromatography-MS (GC-MS) to compare VOC emissions in Pip-deficient (HvALD1 mutants) vs. wild-type plants .
- Air-transfer experiments : Place Pip-treated "sender" plants near receiver plants, then challenge receivers with pathogens (e.g., Blumeria graminis) to quantify defense activation .
- Genetic disruption : Knock out VOC receptors in receiver plants to isolate Pip’s role in signal perception .
Q. How do lysine metabolic pathways influence this compound accumulation in stress responses, and what tools can dissect these interactions?
- Isotope tracing : Use ¹³C-lysine to map flux into Pip under stress (e.g., pathogen infection, salinity).
- Multi-omics integration : Combine transcriptomics (e.g., RNA-seq of lysine/Pip-related genes) with metabolomics to identify regulatory nodes .
- Enzyme kinetics : Compare substrate specificity of ALD1 homologs across species using recombinant protein assays .
Q. Methodological Considerations
- CRISPR/Cas9 design : For plant studies, use dual gRNAs to induce large deletions (e.g., 250 bp in barley HvALD1) for efficient knockout .
- Data normalization : In Pip quantification, normalize to tissue weight and internal standards (e.g., deuterated Pip) to account for extraction variability .
- Controlled environments : Maintain consistent humidity and light in SAR experiments to minimize confounding VOC profiles .
Comparison with Similar Compounds
Comparison with Structurally and Functionally Similar Compounds
Pipecolic Acid vs. Proline
This compound and proline share structural homology as cyclic amino acids but differ in ring size and metabolic origins. While proline is integral to osmoregulation, this compound is critical in systemic acquired resistance (SAR) in plants and microbial secondary metabolism .
This compound vs. N-Hydroxythis compound (NHP)
NHP, a hydroxylated derivative of this compound, is a potent regulator of plant immunity, enhancing salicylic acid (SA)-dependent defenses . Unlike this compound, NHP directly activates defense genes, illustrating how minor structural modifications alter bioactivity .
This compound vs. Sarcosine
L-pipecolic acid oxidase, essential for this compound degradation, shares structural similarity with sarcosine oxidase but exhibits substrate specificity, highlighting functional divergence in amino acid catabolism .
This compound vs. 5-Aminovaleric Acid Betaine (5-AVAB)
5-AVAB, a betaine derivative structurally related to this compound, influences energy metabolism, contrasting with this compound’s roles in defense and secondary metabolism .
Key Research Findings
- Biosynthetic Pathways: this compound is synthesized in microbes via two lysine-derived routes: (1) the P2C pathway (loss of α-amino group) and (2) the P6C pathway (loss of ε-amino group). Fungal species like Penicillium chrysogenum also produce it via α-aminoadipic acid (α-AAA) .
- Enantiomer-Specific Functions: (S)-Pipecolic acid is prevalent in immunosuppressants (e.g., rapamycin), while (R)-pipecolic acid occurs in histone deacetylase inhibitors (e.g., trapoxin A) .
- Enzymatic Engineering: Recombinant E.
Preparation Methods
Enzymatic Resolution and Conversion Techniques
Enzymatic Resolution of Racemic Mixtures
The resolution of DL-pipecolic acid into enantiomerically pure L- or D-forms is a cornerstone of industrial production. A patented method involves sequential resolution with tartaric acid derivatives. For example, treating DL-pipecolic acid with D-tartaric acid at 110°C for four hours selectively crystallizes the L-pipecolic acid-D-tartrate complex, which is subsequently hydrolyzed to yield L-pipecolic acid with >99.5% optical purity . This approach avoids the need for chiral chromatography, making it cost-effective for large-scale synthesis.
Parallel methods employ microbial enzymes for resolution. Aspergillus niger lipase partially resolves DL-pipecolic acid, achieving 93% enantiomeric excess (ee) of (S)-pipecolic acid . However, further purification is required to reach pharmaceutical-grade purity. In contrast, Pseudomonas sp. PA09 utilizes L-lysine as a substrate, converting it directly to L-pipecolic acid via a dehydrogenase pathway with 100% ee .
Direct Enzymatic Conversion of L-Lysine
The cyclodeaminase RapL, identified in the rapamycin biosynthetic pathway, catalyzes the conversion of L-lysine to L-pipecolic acid through a redox-dependent mechanism . This reaction involves the transient oxidation of L-lysine’s α-amine to form Δ¹-piperideine-2-carboxylate, followed by re-reduction to L-pipecolic acid. RapL exhibits a k<sub>cat</sub>/K<sub>m</sub> of 1.2 × 10³ M⁻¹s⁻¹ for L-lysine and requires NAD⁺ as a cofactor . While efficient, this method is primarily employed in microbial systems for secondary metabolite biosynthesis rather than standalone this compound production .
Chemical Synthesis and Resolution Strategies
Racemic Synthesis via Cyclization
This compound is synthesized racemically through the cyclization of δ-chloro-α-aminocaproic acid or its derivatives. For example, ethyl 1-methyl-2-oxocyclopentanecarboxylate undergoes reductive amination to yield 2-methylthis compound, which is subsequently resolved into enantiomers . This method, while versatile for substituted pipecolic acids, requires additional steps to achieve optical purity.
Diastereomeric Salt Formation
Chiral resolution via diastereomeric salt formation remains a widely used technique. The N-carbobenzoxy (Cbz) derivatives of DL-pipecolic acid are treated with quinine or other chiral bases to form separable salts. For instance, the quinine salt of N-Cbz-DL-pipecolic acid preferentially crystallizes the (S)-enantiomer, which is hydrolyzed to yield L-pipecolic acid . Modifying the protecting group (e.g., using 4-phenylbenzyloxycarbonyl) enhances selectivity for cis-6-methylthis compound derivatives .
Table 1: Resolution Efficiency of this compound Derivatives
Substrate | Resolving Agent | Yield (%) | ee (%) | Reference |
---|---|---|---|---|
DL-Pipecolic acid | D-Tartaric acid | 65 | >99.5 | |
N-Cbz-DL-Pipecolic acid | Quinine | 45 | 85–90 | |
6-Methyl-DL-pipecolic acid | 4-Phenylbenzyloxycarbonyl | 71 | 99.5 |
Biosynthetic Pathways in Microorganisms
Lysine Cyclodeaminase Pathways
Microbes such as Streptomyces hygroscopicus (rapamycin producer) and Mycobacterium tuberculosis utilize lysine cyclodeaminases (LCDs) to convert L-lysine directly to L-pipecolic acid . These enzymes are critical for incorporating this compound into macrolactone antibiotics. RapL, a characterized LCD, operates via an NAD⁺-dependent mechanism, retaining the α-hydrogen of L-lysine while eliminating the α-amine .
Alternative Microbial Routes
Some bacteria employ a two-step pathway: L-lysine is first oxidized to Δ¹-piperideine-6-carboxylic acid by lysine oxidase, followed by non-enzymatic cyclization to this compound . This route is less efficient than LCD-mediated pathways but provides flexibility in organisms lacking dedicated cyclodeaminases.
Industrial-Scale Production and Optimization
Crystallization and Purification Techniques
Final purification of L-pipecolic acid involves recrystallization from ethyl acetate or acetone/water mixtures. For example, dissolving ropivacaine free base in hot acetone/water (1:2 v/v) and cooling to 0°C yields the hydrochloride monohydrate with 93% recovery and 5.5% water content . X-ray diffraction patterns (Figs. 1–3 in ) confirm crystalline purity.
Table 2: Industrial Process Parameters for this compound Derivatives
Step | Conditions | Yield (%) | Purity (%) |
---|---|---|---|
L-Pipecolic acid resolution | D-Tartaric acid, 110°C, 4h | 65 | >99.5 |
L-N-Pipecolyxylidide synthesis | PCl₅, –30°C, 2h | 71 | 99.5 |
Ropivacaine HCl crystallization | Acetone/water, 0°C | 93 | 99.8 |
Properties
IUPAC Name |
piperidine-2-carboxylic acid | |
---|---|---|
Source | PubChem | |
URL | https://pubchem.ncbi.nlm.nih.gov | |
Description | Data deposited in or computed by PubChem | |
InChI |
InChI=1S/C6H11NO2/c8-6(9)5-3-1-2-4-7-5/h5,7H,1-4H2,(H,8,9) | |
Source | PubChem | |
URL | https://pubchem.ncbi.nlm.nih.gov | |
Description | Data deposited in or computed by PubChem | |
InChI Key |
HXEACLLIILLPRG-UHFFFAOYSA-N | |
Source | PubChem | |
URL | https://pubchem.ncbi.nlm.nih.gov | |
Description | Data deposited in or computed by PubChem | |
Canonical SMILES |
C1CCNC(C1)C(=O)O | |
Source | PubChem | |
URL | https://pubchem.ncbi.nlm.nih.gov | |
Description | Data deposited in or computed by PubChem | |
Molecular Formula |
C6H11NO2 | |
Source | PubChem | |
URL | https://pubchem.ncbi.nlm.nih.gov | |
Description | Data deposited in or computed by PubChem | |
Related CAS |
69470-51-5 (mono-potassium salt), 15862-86-9 (hydrochloride salt/solvate) | |
Record name | Pipecolic acid | |
Source | ChemIDplus | |
URL | https://pubchem.ncbi.nlm.nih.gov/substance/?source=chemidplus&sourceid=0000535751 | |
Description | ChemIDplus is a free, web search system that provides access to the structure and nomenclature authority files used for the identification of chemical substances cited in National Library of Medicine (NLM) databases, including the TOXNET system. | |
DSSTOX Substance ID |
DTXSID40862144 | |
Record name | 2-Piperidinecarboxylic acid | |
Source | EPA DSSTox | |
URL | https://comptox.epa.gov/dashboard/DTXSID40862144 | |
Description | DSSTox provides a high quality public chemistry resource for supporting improved predictive toxicology. | |
Molecular Weight |
129.16 g/mol | |
Source | PubChem | |
URL | https://pubchem.ncbi.nlm.nih.gov | |
Description | Data deposited in or computed by PubChem | |
Physical Description |
Solid | |
Record name | Pipecolic acid | |
Source | Human Metabolome Database (HMDB) | |
URL | http://www.hmdb.ca/metabolites/HMDB0000070 | |
Description | The Human Metabolome Database (HMDB) is a freely available electronic database containing detailed information about small molecule metabolites found in the human body. | |
Explanation | HMDB is offered to the public as a freely available resource. Use and re-distribution of the data, in whole or in part, for commercial purposes requires explicit permission of the authors and explicit acknowledgment of the source material (HMDB) and the original publication (see the HMDB citing page). We ask that users who download significant portions of the database cite the HMDB paper in any resulting publications. | |
Solubility |
314 mg/mL | |
Record name | Pipecolic acid | |
Source | Human Metabolome Database (HMDB) | |
URL | http://www.hmdb.ca/metabolites/HMDB0000070 | |
Description | The Human Metabolome Database (HMDB) is a freely available electronic database containing detailed information about small molecule metabolites found in the human body. | |
Explanation | HMDB is offered to the public as a freely available resource. Use and re-distribution of the data, in whole or in part, for commercial purposes requires explicit permission of the authors and explicit acknowledgment of the source material (HMDB) and the original publication (see the HMDB citing page). We ask that users who download significant portions of the database cite the HMDB paper in any resulting publications. | |
CAS No. |
535-75-1, 3105-95-1, 4043-87-2 | |
Record name | Pipecolic acid | |
Source | CAS Common Chemistry | |
URL | https://commonchemistry.cas.org/detail?cas_rn=535-75-1 | |
Description | CAS Common Chemistry is an open community resource for accessing chemical information. Nearly 500,000 chemical substances from CAS REGISTRY cover areas of community interest, including common and frequently regulated chemicals, and those relevant to high school and undergraduate chemistry classes. This chemical information, curated by our expert scientists, is provided in alignment with our mission as a division of the American Chemical Society. | |
Explanation | The data from CAS Common Chemistry is provided under a CC-BY-NC 4.0 license, unless otherwise stated. | |
Record name | Pipecolic acid | |
Source | ChemIDplus | |
URL | https://pubchem.ncbi.nlm.nih.gov/substance/?source=chemidplus&sourceid=0000535751 | |
Description | ChemIDplus is a free, web search system that provides access to the structure and nomenclature authority files used for the identification of chemical substances cited in National Library of Medicine (NLM) databases, including the TOXNET system. | |
Record name | Pipecolic acid | |
Source | DTP/NCI | |
URL | https://dtp.cancer.gov/dtpstandard/servlet/dwindex?searchtype=NSC&outputformat=html&searchlist=93089 | |
Description | The NCI Development Therapeutics Program (DTP) provides services and resources to the academic and private-sector research communities worldwide to facilitate the discovery and development of new cancer therapeutic agents. | |
Explanation | Unless otherwise indicated, all text within NCI products is free of copyright and may be reused without our permission. Credit the National Cancer Institute as the source. | |
Record name | Pipecolic acid | |
Source | DTP/NCI | |
URL | https://dtp.cancer.gov/dtpstandard/servlet/dwindex?searchtype=NSC&outputformat=html&searchlist=17125 | |
Description | The NCI Development Therapeutics Program (DTP) provides services and resources to the academic and private-sector research communities worldwide to facilitate the discovery and development of new cancer therapeutic agents. | |
Explanation | Unless otherwise indicated, all text within NCI products is free of copyright and may be reused without our permission. Credit the National Cancer Institute as the source. | |
Record name | 2-Piperidinecarboxylic acid | |
Source | EPA Chemicals under the TSCA | |
URL | https://www.epa.gov/chemicals-under-tsca | |
Description | EPA Chemicals under the Toxic Substances Control Act (TSCA) collection contains information on chemicals and their regulations under TSCA, including non-confidential content from the TSCA Chemical Substance Inventory and Chemical Data Reporting. | |
Record name | 2-Piperidinecarboxylic acid | |
Source | EPA DSSTox | |
URL | https://comptox.epa.gov/dashboard/DTXSID40862144 | |
Description | DSSTox provides a high quality public chemistry resource for supporting improved predictive toxicology. | |
Record name | DL-piperidine-2-carboxylic acid | |
Source | European Chemicals Agency (ECHA) | |
URL | https://echa.europa.eu/substance-information/-/substanceinfo/100.021.580 | |
Description | The European Chemicals Agency (ECHA) is an agency of the European Union which is the driving force among regulatory authorities in implementing the EU's groundbreaking chemicals legislation for the benefit of human health and the environment as well as for innovation and competitiveness. | |
Explanation | Use of the information, documents and data from the ECHA website is subject to the terms and conditions of this Legal Notice, and subject to other binding limitations provided for under applicable law, the information, documents and data made available on the ECHA website may be reproduced, distributed and/or used, totally or in part, for non-commercial purposes provided that ECHA is acknowledged as the source: "Source: European Chemicals Agency, http://echa.europa.eu/". Such acknowledgement must be included in each copy of the material. ECHA permits and encourages organisations and individuals to create links to the ECHA website under the following cumulative conditions: Links can only be made to webpages that provide a link to the Legal Notice page. | |
Record name | Piperidine-2-carboxylic acid | |
Source | European Chemicals Agency (ECHA) | |
URL | https://echa.europa.eu/substance-information/-/substanceinfo/100.007.835 | |
Description | The European Chemicals Agency (ECHA) is an agency of the European Union which is the driving force among regulatory authorities in implementing the EU's groundbreaking chemicals legislation for the benefit of human health and the environment as well as for innovation and competitiveness. | |
Explanation | Use of the information, documents and data from the ECHA website is subject to the terms and conditions of this Legal Notice, and subject to other binding limitations provided for under applicable law, the information, documents and data made available on the ECHA website may be reproduced, distributed and/or used, totally or in part, for non-commercial purposes provided that ECHA is acknowledged as the source: "Source: European Chemicals Agency, http://echa.europa.eu/". Such acknowledgement must be included in each copy of the material. ECHA permits and encourages organisations and individuals to create links to the ECHA website under the following cumulative conditions: Links can only be made to webpages that provide a link to the Legal Notice page. | |
Record name | PIPECOLIC ACID | |
Source | FDA Global Substance Registration System (GSRS) | |
URL | https://gsrs.ncats.nih.gov/ginas/app/beta/substances/H254GW7PVV | |
Description | The FDA Global Substance Registration System (GSRS) enables the efficient and accurate exchange of information on what substances are in regulated products. Instead of relying on names, which vary across regulatory domains, countries, and regions, the GSRS knowledge base makes it possible for substances to be defined by standardized, scientific descriptions. | |
Explanation | Unless otherwise noted, the contents of the FDA website (www.fda.gov), both text and graphics, are not copyrighted. They are in the public domain and may be republished, reprinted and otherwise used freely by anyone without the need to obtain permission from FDA. Credit to the U.S. Food and Drug Administration as the source is appreciated but not required. | |
Record name | Pipecolic acid | |
Source | Human Metabolome Database (HMDB) | |
URL | http://www.hmdb.ca/metabolites/HMDB0000070 | |
Description | The Human Metabolome Database (HMDB) is a freely available electronic database containing detailed information about small molecule metabolites found in the human body. | |
Explanation | HMDB is offered to the public as a freely available resource. Use and re-distribution of the data, in whole or in part, for commercial purposes requires explicit permission of the authors and explicit acknowledgment of the source material (HMDB) and the original publication (see the HMDB citing page). We ask that users who download significant portions of the database cite the HMDB paper in any resulting publications. | |
Melting Point |
264 °C | |
Record name | Pipecolic acid | |
Source | Human Metabolome Database (HMDB) | |
URL | http://www.hmdb.ca/metabolites/HMDB0000070 | |
Description | The Human Metabolome Database (HMDB) is a freely available electronic database containing detailed information about small molecule metabolites found in the human body. | |
Explanation | HMDB is offered to the public as a freely available resource. Use and re-distribution of the data, in whole or in part, for commercial purposes requires explicit permission of the authors and explicit acknowledgment of the source material (HMDB) and the original publication (see the HMDB citing page). We ask that users who download significant portions of the database cite the HMDB paper in any resulting publications. | |
Retrosynthesis Analysis
AI-Powered Synthesis Planning: Our tool employs the Template_relevance Pistachio, Template_relevance Bkms_metabolic, Template_relevance Pistachio_ringbreaker, Template_relevance Reaxys, Template_relevance Reaxys_biocatalysis model, leveraging a vast database of chemical reactions to predict feasible synthetic routes.
One-Step Synthesis Focus: Specifically designed for one-step synthesis, it provides concise and direct routes for your target compounds, streamlining the synthesis process.
Accurate Predictions: Utilizing the extensive PISTACHIO, BKMS_METABOLIC, PISTACHIO_RINGBREAKER, REAXYS, REAXYS_BIOCATALYSIS database, our tool offers high-accuracy predictions, reflecting the latest in chemical research and data.
Strategy Settings
Precursor scoring | Relevance Heuristic |
---|---|
Min. plausibility | 0.01 |
Model | Template_relevance |
Template Set | Pistachio/Bkms_metabolic/Pistachio_ringbreaker/Reaxys/Reaxys_biocatalysis |
Top-N result to add to graph | 6 |
Feasible Synthetic Routes
Disclaimer and Information on In-Vitro Research Products
Please be aware that all articles and product information presented on BenchChem are intended solely for informational purposes. The products available for purchase on BenchChem are specifically designed for in-vitro studies, which are conducted outside of living organisms. In-vitro studies, derived from the Latin term "in glass," involve experiments performed in controlled laboratory settings using cells or tissues. It is important to note that these products are not categorized as medicines or drugs, and they have not received approval from the FDA for the prevention, treatment, or cure of any medical condition, ailment, or disease. We must emphasize that any form of bodily introduction of these products into humans or animals is strictly prohibited by law. It is essential to adhere to these guidelines to ensure compliance with legal and ethical standards in research and experimentation.