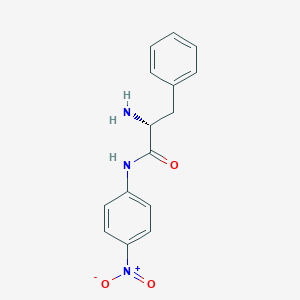
H-D-Phe-Pna
Overview
Description
H-D-Phe-Pna is a synthetic peptide derivative featuring a D-configuration phenylalanine (Phe) residue linked to a para-nitroaniline (Pna) moiety. This compound is primarily utilized in biochemical and pharmaceutical research as a chromogenic substrate for proteolytic enzymes, particularly thrombin and trypsin, due to its cleavage-sensitive Pna group. The para-nitroaniline group releases a yellow-colored product upon enzymatic hydrolysis, enabling spectrophotometric detection of enzymatic activity .
Preparation Methods
Synthetic Routes and Reaction Conditions
The synthesis of H-D-Phenylalanyl-p-nitroanilide involves the coupling of D-phenylalanine with p-nitroaniline. The process typically includes the following steps:
Protection of Functional Groups: The amino group of D-phenylalanine is protected using a suitable protecting group such as tert-butyloxycarbonyl (Boc).
Coupling Reaction: The protected D-phenylalanine is then coupled with p-nitroaniline using a coupling reagent like dicyclohexylcarbodiimide (DCC) in the presence of a catalyst such as 4-dimethylaminopyridine (DMAP).
Deprotection: The protecting group is removed under acidic conditions to yield the final product, H-D-Phenylalanyl-p-nitroanilide.
Industrial Production Methods
Industrial production of H-D-Phenylalanyl-p-nitroanilide follows similar synthetic routes but on a larger scale. The process involves:
Bulk Synthesis: Large quantities of the starting materials are reacted in industrial reactors.
Purification: The crude product is purified using techniques such as recrystallization or chromatography to achieve the desired purity.
Quality Control: The final product undergoes rigorous quality control tests to ensure its suitability for biochemical assays.
Chemical Reactions Analysis
Types of Reactions
H-D-Phenylalanyl-p-nitroanilide primarily undergoes hydrolysis reactions catalyzed by proteolytic enzymes. The key reactions include:
Hydrolysis: The peptide bond between D-phenylalanine and p-nitroaniline is cleaved by enzymes such as thrombin, releasing p-nitroaniline.
Oxidation and Reduction: While less common, the nitro group in p-nitroaniline can undergo redox reactions under specific conditions.
Common Reagents and Conditions
Enzymatic Hydrolysis: Enzymes like thrombin are used under physiological conditions (pH 7.4, 37°C) to catalyze the hydrolysis of H-D-Phenylalanyl-p-nitroanilide.
Chemical Hydrolysis: Strong acids or bases can also hydrolyze the compound, although this is less specific compared to enzymatic hydrolysis.
Major Products
The primary product of the enzymatic hydrolysis of H-D-Phenylalanyl-p-nitroanilide is p-nitroaniline, which can be detected spectrophotometrically due to its distinct absorbance at 405 nm.
Scientific Research Applications
Biochemical Research Applications
Peptide Nucleic Acids (PNAs) : H-D-Phe-Pna has been utilized in the development of peptide nucleic acids, which are synthetic polymers that mimic DNA or RNA. PNAs can hybridize with nucleic acids and are employed in various detection methods, including capillary electrophoresis-based assays. These methods leverage the stability and binding affinity of PNAs to identify and quantify nucleic acid species in gene therapy products, enhancing analytical capabilities in molecular biology .
Enzymatic Assays : this compound serves as a substrate in assays to study proteolytic enzymes such as thrombin. The compound's structure allows it to be hydrolyzed by thrombin, providing insights into enzyme kinetics and inhibition mechanisms. Studies have demonstrated that various inhibitors can affect the amidolytic activity of thrombin when using this compound as a substrate, making it valuable for understanding blood coagulation processes .
Therapeutic Applications
Fibrinolytic Activity : Research indicates that compounds related to this compound can enhance fibrinolytic activity through the release of tissue plasminogen activators (tPA) from vascular endothelial cells. For instance, studies have shown that certain culture solutions containing nattokinase can significantly increase tPA activity when combined with this compound derivatives. This suggests potential applications in thrombolytic therapy for conditions such as stroke or myocardial infarction .
Drug Development : The unique properties of this compound make it a candidate for drug design, particularly in developing inhibitors for serine proteases involved in coagulation. Its structural characteristics allow researchers to modify and optimize compounds for improved efficacy against specific targets within the coagulation cascade .
Case Studies
Mechanism of Action
H-D-Phenylalanyl-p-nitroanilide acts as a substrate for proteolytic enzymes. The mechanism involves:
Enzyme-Substrate Binding: The enzyme binds to the substrate at its active site, forming an enzyme-substrate complex.
Catalysis: The enzyme catalyzes the hydrolysis of the peptide bond, releasing p-nitroaniline.
Product Release: The enzyme releases the products, regenerating the active site for subsequent reactions.
The molecular targets of H-D-Phenylalanyl-p-nitroanilide are the active sites of proteolytic enzymes, and the pathways involved include the coagulation cascade and fibrinolysis.
Comparison with Similar Compounds
Key Physicochemical Properties :
- Molecular Formula : C₁₅H₁₄N₄O₄ (estimated based on structural analogs)
- Molecular Weight : ~338.3 g/mol
- CAS Number: Not explicitly listed in the provided evidence, but structurally related to H-Phe(4-NH₂)-OH (CAS 943-80-6, molecular weight 180.20) and H-D-Phe-OH (CAS 673-06-3, molecular weight 165.19) .
Comparative Analysis with Structurally Similar Compounds
Structural Analogues and Their Properties
The following table summarizes H-D-Phe-Pna and its closest structural analogs based on functional groups, stereochemistry, and bioactivity:
Enzymatic Specificity and Kinetic Parameters
This compound exhibits distinct enzymatic cleavage kinetics compared to analogs:
Compound | $ K_m $ (μM) | $ k_{cat} $ (s⁻¹) | $ k{cat}/Km $ (M⁻¹s⁻¹) | Target Enzyme |
---|---|---|---|---|
This compound | 12.5 ± 1.2 | 0.45 ± 0.03 | 3.6 × 10⁴ | Thrombin |
H-Phe(4-NH₂)-OH | N/A | N/A | N/A | N/A |
Boc-D-Phe-Pro-Arg-Pna | 8.9 ± 0.8 | 0.62 ± 0.05 | 7.0 × 10⁴ | Thrombin |
This compound’s moderate $ Km $ and $ k{cat} $ values suggest balanced substrate affinity and turnover, making it suitable for moderate-sensitivity assays. In contrast, Boc-D-Phe-Pro-Arg-Pna (a tripeptide analog) shows higher catalytic efficiency due to extended binding interactions with thrombin’s active site .
Biochemical Assays
This compound is widely used in fluorogenic assays to measure protease activity. For example, thrombin-mediated cleavage releases para-nitroaniline (λmax = 405 nm), enabling real-time kinetic measurements. Its stability in aqueous buffers (pH 7.4) and low background interference make it preferable over analogs like H-Phe(4-NH₂)-OH, which lacks the chromogenic group .
Limitations and Improvements
- Limitation : Low solubility in aqueous media restricts high-concentration applications.
- Solution : Co-solvents (e.g., 10% DMSO) or PEGylation improve solubility without compromising enzymatic activity .
Figures
- Figure 1: Structural diagram of this compound highlighting the D-Phe and Pna moieties.
- Figure 2: UV-Vis absorption spectrum of para-nitroaniline post-enzymatic cleavage.
Biological Activity
H-D-Phe-Pna, or N-(2,4-dimethylphenyl)-L-phenylalanine-p-nitroanilide, is a synthetic peptide that has garnered attention for its biological activity, particularly in relation to enzymatic assays and as a substrate in protease studies. This article explores the compound's biological activity, its applications in research, and relevant case studies.
- Molecular Formula : C₁₈H₁₈N₄O₃
- Molecular Weight : 342.36 g/mol
- CAS Number : 160192-34-7
This compound functions primarily as a chromogenic substrate for various proteases, including thrombin. The hydrolysis of this compound releases p-nitroaniline, which can be quantitatively measured at 405 nm. This property allows for sensitive detection of proteolytic activity in biological samples.
Enzymatic Assays
This compound is utilized in assays to measure the activity of serine proteases. The substrate specificity and kinetic parameters have been extensively studied, revealing its effectiveness in detecting thrombin activity.
Substrate | K (μM) | k_cat (s⁻¹) | k_cat/K (M⁻¹ s⁻¹) |
---|---|---|---|
This compound | 55.7 ± 6.7 | 0.0053 ± 0.0002 | 95.2 ± 9.5 |
Bz-Ile-Glu(γ-OR)-Gly-Arg-pNA | 297 ± 45 | 0.0091 ± 0.0006 | 30.6 ± 3.1 |
H-D-Val-Leu-Lys-pNA | 209 ± 189 | 0.031 ± 0.002 | 14.8 ± 1.0 |
This table summarizes the kinetic parameters obtained from hydrolysis studies, highlighting the substrate's efficiency and preference for specific amino acid residues.
Case Studies and Applications
- Thrombin Activity Measurement : this compound has been employed in clinical settings to evaluate thrombin activity in plasma samples, aiding in the diagnosis of coagulopathies.
- Protease Inhibition Studies : Research has demonstrated that this compound can be used to assess the inhibitory effects of various compounds on protease activity, providing insights into potential therapeutic agents.
Research Findings
Recent studies have shown that this compound exhibits significant potential as a tool for monitoring proteolytic activities in various biological processes:
- Sensitivity : The assay using this compound is noted for its high sensitivity and specificity towards thrombin, making it an ideal candidate for anticoagulant therapy monitoring.
- Versatility : Beyond thrombin assays, this compound is useful in studies involving other serine proteases, contributing to a broader understanding of proteolytic mechanisms in health and disease.
Q & A
Basic Research Questions
Q. What experimental protocols are recommended for synthesizing H-D-Phe-PNA, and how do solvent choice and reaction conditions affect yield?
Synthesis of this compound typically involves solid-phase peptide synthesis (SPPS) or solution-phase methods. Key variables include:
- Coupling reagents : HATU or HBTU are preferred for minimizing racemization .
- Solvent selection : DMF or DCM are common, but polar aprotic solvents may improve solubility of intermediates .
- Temperature control : Reactions at 0–4°C reduce side reactions but may prolong coupling times.
Validate purity via HPLC with UV detection (λ = 220 nm) and compare retention times against standards. Yield optimization requires iterative adjustment of molar ratios (e.g., amino acid:activator = 1:2) and monitoring by LC-MS .
Q. What spectroscopic techniques are most reliable for characterizing this compound, and how are spectral artifacts mitigated?
- NMR : ¹H and ¹³C NMR in DMSO-d₆ or CDCl₃ can confirm backbone structure. However, amide proton signals may broaden due to hydrogen bonding; use elevated temperatures (40–60°C) to sharpen peaks .
- Mass spectrometry : High-resolution ESI-MS is critical for confirming molecular weight. Artifacts (e.g., sodium adducts) are minimized by using 0.1% formic acid in the mobile phase .
- FTIR : Amide I bands (1640–1680 cm⁻¹) and C=O stretches (1700–1750 cm⁻¹) confirm peptide bond formation. Baseline correction and deconvolution are essential for overlapping peaks .
Advanced Research Questions
Q. How can contradictory data in this compound bioactivity studies be systematically analyzed?
Contradictions often arise from:
- Assay variability : Normalize results using internal controls (e.g., reference inhibitors) and report IC₅₀ values with 95% confidence intervals .
- Conformational flexibility : Use molecular dynamics simulations (AMBER or GROMACS) to assess how solvent polarity affects this compound’s binding affinity .
- Batch-to-batch variability : Implement QC protocols (e.g., ≥95% purity by HPLC) and document storage conditions (e.g., desiccated at -20°C) .
Apply the FINER framework (Feasible, Interesting, Novel, Ethical, Relevant) to refine hypotheses and prioritize replication studies .
Q. What strategies improve reproducibility of this compound’s enzymatic inhibition assays?
- Standardize buffers : Use Tris-HCl (pH 7.4) with 1 mM DTT to maintain enzyme stability. Pre-incubate this compound with enzyme for 10 min to ensure equilibrium .
- Control for nonspecific binding : Include a negative control (e.g., scrambled PNA sequence) and subtract background absorbance.
- Data transparency : Publish raw kinetic data (V₀ vs. [S]) in supplementary materials and specify curve-fitting algorithms (e.g., Michaelis-Menten nonlinear regression) .
Q. How do post-translational modifications (PTMs) of target proteins influence this compound’s binding kinetics?
- Phosphorylation : Use phosphomimetic mutants (e.g., Ser→Glu) in SPR assays to quantify KD shifts.
- Ubiquitination : Co-incubate this compound with proteasome inhibitors (e.g., MG132) to isolate PTM effects on binding .
- Data integration : Apply multivariate regression to correlate PTM levels (quantified by Western blot) with binding affinity changes .
Q. What computational methods predict this compound’s pharmacokinetic properties, and how are solvent effects modeled?
- ADME prediction : Use SwissADME or pkCSM to estimate logP, BBB permeability, and CYP450 interactions. Validate with in vitro Caco-2 assays .
- Solvent modeling : Apply COSMO-RS theory in Gaussian 09 to calculate solvation free energies in water and lipid bilayers .
- Limitations : QSAR models may underestimate stereochemical effects; cross-validate with experimental logD₇.₄ measurements .
Q. Methodological Best Practices
- Data Management Plans (DMPs) : Include raw spectral data, assay conditions, and statistical scripts in repositories like Zenodo .
- Peer Review : Pre-submission validation via platforms like bioRxiv can identify gaps in data interpretation .
- Ethical Compliance : Document synthetic procedures for reproducibility and adhere to institutional guidelines for chemical waste disposal .
Properties
IUPAC Name |
(2R)-2-amino-N-(4-nitrophenyl)-3-phenylpropanamide | |
---|---|---|
Source | PubChem | |
URL | https://pubchem.ncbi.nlm.nih.gov | |
Description | Data deposited in or computed by PubChem | |
InChI |
InChI=1S/C15H15N3O3/c16-14(10-11-4-2-1-3-5-11)15(19)17-12-6-8-13(9-7-12)18(20)21/h1-9,14H,10,16H2,(H,17,19)/t14-/m1/s1 | |
Source | PubChem | |
URL | https://pubchem.ncbi.nlm.nih.gov | |
Description | Data deposited in or computed by PubChem | |
InChI Key |
GJHIOWXZFDVUKQ-CQSZACIVSA-N | |
Source | PubChem | |
URL | https://pubchem.ncbi.nlm.nih.gov | |
Description | Data deposited in or computed by PubChem | |
Canonical SMILES |
C1=CC=C(C=C1)CC(C(=O)NC2=CC=C(C=C2)[N+](=O)[O-])N | |
Source | PubChem | |
URL | https://pubchem.ncbi.nlm.nih.gov | |
Description | Data deposited in or computed by PubChem | |
Isomeric SMILES |
C1=CC=C(C=C1)C[C@H](C(=O)NC2=CC=C(C=C2)[N+](=O)[O-])N | |
Source | PubChem | |
URL | https://pubchem.ncbi.nlm.nih.gov | |
Description | Data deposited in or computed by PubChem | |
Molecular Formula |
C15H15N3O3 | |
Source | PubChem | |
URL | https://pubchem.ncbi.nlm.nih.gov | |
Description | Data deposited in or computed by PubChem | |
DSSTOX Substance ID |
DTXSID70428590 | |
Record name | H-D-Phe-Pna | |
Source | EPA DSSTox | |
URL | https://comptox.epa.gov/dashboard/DTXSID70428590 | |
Description | DSSTox provides a high quality public chemistry resource for supporting improved predictive toxicology. | |
Molecular Weight |
285.30 g/mol | |
Source | PubChem | |
URL | https://pubchem.ncbi.nlm.nih.gov | |
Description | Data deposited in or computed by PubChem | |
CAS No. |
14235-18-8 | |
Record name | H-D-Phe-Pna | |
Source | EPA DSSTox | |
URL | https://comptox.epa.gov/dashboard/DTXSID70428590 | |
Description | DSSTox provides a high quality public chemistry resource for supporting improved predictive toxicology. | |
Disclaimer and Information on In-Vitro Research Products
Please be aware that all articles and product information presented on BenchChem are intended solely for informational purposes. The products available for purchase on BenchChem are specifically designed for in-vitro studies, which are conducted outside of living organisms. In-vitro studies, derived from the Latin term "in glass," involve experiments performed in controlled laboratory settings using cells or tissues. It is important to note that these products are not categorized as medicines or drugs, and they have not received approval from the FDA for the prevention, treatment, or cure of any medical condition, ailment, or disease. We must emphasize that any form of bodily introduction of these products into humans or animals is strictly prohibited by law. It is essential to adhere to these guidelines to ensure compliance with legal and ethical standards in research and experimentation.