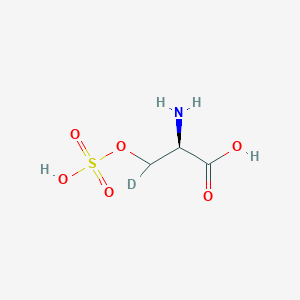
O-Sulfo-D-serine
Overview
Description
O-Sulfo-D-serine (O-SDS) is a naturally occurring sulfated amino acid that has been found to play important roles in various biological processes. It was first identified in the brain tissue of rats and has since been found in other tissues such as the liver, kidney, and lung. O-SDS is synthesized by the enzyme sulfotransferase, which transfers a sulfate group from a donor molecule to the hydroxyl group of serine.
Scientific Research Applications
Collision-Induced Dissociation Studies
O-Sulfo-D-serine has been studied in the context of collision-induced dissociation (CID). Lucas and Barnes (2020) used direct dynamics simulations and density functional theory (DFT) calculations to explore the effects of O-sulfonation on the CID of serine. They found that specific peaks in the theoretical mass spectrum were directly related to the sulfo group, indicating significant structural impacts due to sulfonation. This research has implications for understanding the molecular behavior of O-sulfo-D-serine under collision conditions (Lucas & Barnes, 2020).
Enzyme Interaction Studies
The interaction of serine acetyltransferase with O-acetylserine sulfhydrylase, a related enzyme, has been studied to understand the sulfur assimilation pathway in bacteria and plants. Campanini et al. (2005) investigated this interaction and found that it influenced the enzyme's catalytic activity, suggesting a regulatory mechanism involving serine derivatives in the cysteine biosynthetic pathway (Campanini et al., 2005).
Neurotoxicity Studies
Wang et al. (2019) researched the role of astrocytes-derived D-serine in PFOS-induced neurotoxicity. They found that alterations in D-serine were mediated by molecules involved in D-serine synthesis and metabolism, suggesting a critical role of serine derivatives in neurotoxic responses (Wang et al., 2019).
Sulfate Assimilation Regulation
Neuenschwander et al. (1991) studied the effect of O-acetyl-L-serine on sulfate assimilation in Lemna minor L. They found that O-acetyl-L-serine had a regulatory effect on sulfate assimilation, indicating its importance in biochemical pathways involving sulfur (Neuenschwander et al., 1991).
Enzyme Inhibition Studies
Carvalho et al. (2020) developed 3-oxo-β-sultam inhibitors that interacted with serine hydrolases. Their study highlights the potential of sulfonated compounds, including those derived from serine, in the development of enzyme inhibitors (Carvalho et al., 2020).
Spectroscopic Characterization
Paciotti et al. (2015) conducted a study on the O-sulfation of L-serine, revealing the structural and stability effects of protonation and deprotonation on sulfated serine ions. This research provides insights into the molecular characteristics of sulfated amino acids, including O-sulfo-D-serine (Paciotti et al., 2015).
Enzymatic Reactions
Winum et al. (2006) discussed the therapeutic potential of sulfamides as enzyme inhibitors, highlighting the biological significance of sulfonated compounds derived from amino acids like serine (Winum et al., 2006).
Polypeptide Transformation
Fasman (1960) demonstrated that concentrated sulfuric acid could convert poly-DL-serine to a polyester, showing the chemical transformability of serine compounds under specific conditions (Fasman, 1960).
Sulfhydrylation Reaction Catalysis
Mino and Ishikawa (2003) studied the substrate specificity of O-acetylserine sulfhydrylase from Aeropyrum pernix K1, which revealed insights into the enzymatic processing of serine derivatives in hyperthermophilic archaea (Mino & Ishikawa, 2003).
Protein Sulfonation Studies
Medzihradszky et al. (2004) described protein sulfonation on serine and threonine residues in various organisms, indicating a role for this modification in protein assembly and signaling. This study sheds light on the broader implications of serine sulfonation in biological systems (Medzihradszky et al., 2004).
properties
IUPAC Name |
(2R)-2-amino-3-sulfooxypropanoic acid | |
---|---|---|
Source | PubChem | |
URL | https://pubchem.ncbi.nlm.nih.gov | |
Description | Data deposited in or computed by PubChem | |
InChI |
InChI=1S/C3H7NO6S/c4-2(3(5)6)1-10-11(7,8)9/h2H,1,4H2,(H,5,6)(H,7,8,9)/t2-/m1/s1 | |
Source | PubChem | |
URL | https://pubchem.ncbi.nlm.nih.gov | |
Description | Data deposited in or computed by PubChem | |
InChI Key |
LFZGUGJDVUUGLK-UWTATZPHSA-N | |
Source | PubChem | |
URL | https://pubchem.ncbi.nlm.nih.gov | |
Description | Data deposited in or computed by PubChem | |
Canonical SMILES |
C(C(C(=O)O)N)OS(=O)(=O)O | |
Source | PubChem | |
URL | https://pubchem.ncbi.nlm.nih.gov | |
Description | Data deposited in or computed by PubChem | |
Isomeric SMILES |
C([C@H](C(=O)O)N)OS(=O)(=O)O | |
Source | PubChem | |
URL | https://pubchem.ncbi.nlm.nih.gov | |
Description | Data deposited in or computed by PubChem | |
Molecular Formula |
C3H7NO6S | |
Source | PubChem | |
URL | https://pubchem.ncbi.nlm.nih.gov | |
Description | Data deposited in or computed by PubChem | |
Molecular Weight |
185.16 g/mol | |
Source | PubChem | |
URL | https://pubchem.ncbi.nlm.nih.gov | |
Description | Data deposited in or computed by PubChem | |
Product Name |
(2R)-2-amino-3-sulfooxypropanoic acid |
Retrosynthesis Analysis
AI-Powered Synthesis Planning: Our tool employs the Template_relevance Pistachio, Template_relevance Bkms_metabolic, Template_relevance Pistachio_ringbreaker, Template_relevance Reaxys, Template_relevance Reaxys_biocatalysis model, leveraging a vast database of chemical reactions to predict feasible synthetic routes.
One-Step Synthesis Focus: Specifically designed for one-step synthesis, it provides concise and direct routes for your target compounds, streamlining the synthesis process.
Accurate Predictions: Utilizing the extensive PISTACHIO, BKMS_METABOLIC, PISTACHIO_RINGBREAKER, REAXYS, REAXYS_BIOCATALYSIS database, our tool offers high-accuracy predictions, reflecting the latest in chemical research and data.
Strategy Settings
Precursor scoring | Relevance Heuristic |
---|---|
Min. plausibility | 0.01 |
Model | Template_relevance |
Template Set | Pistachio/Bkms_metabolic/Pistachio_ringbreaker/Reaxys/Reaxys_biocatalysis |
Top-N result to add to graph | 6 |
Feasible Synthetic Routes
Disclaimer and Information on In-Vitro Research Products
Please be aware that all articles and product information presented on BenchChem are intended solely for informational purposes. The products available for purchase on BenchChem are specifically designed for in-vitro studies, which are conducted outside of living organisms. In-vitro studies, derived from the Latin term "in glass," involve experiments performed in controlled laboratory settings using cells or tissues. It is important to note that these products are not categorized as medicines or drugs, and they have not received approval from the FDA for the prevention, treatment, or cure of any medical condition, ailment, or disease. We must emphasize that any form of bodily introduction of these products into humans or animals is strictly prohibited by law. It is essential to adhere to these guidelines to ensure compliance with legal and ethical standards in research and experimentation.