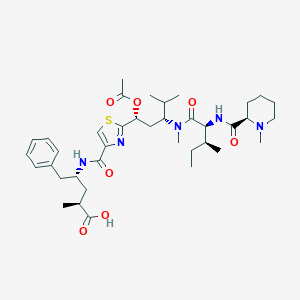
Tubulysin M
Overview
Description
Tubulysin M is a highly potent microtubule inhibitor belonging to the tubulysin family, a group of natural products isolated from myxobacteria. Its mechanism involves binding to the β-subunit of tubulin, disrupting microtubule dynamics, and inducing apoptosis in cancer cells . With an IC50 of 0.02 nM in cancer cell lines, it is among the most cytotoxic compounds known, making it a promising payload for antibody-drug conjugates (ADCs) . Structural features critical to its activity include the C11 acetate group, which stabilizes interactions with tubulin, and the tetrapeptide backbone containing unique residues like tubuphenylalanine and tubuvaline .
Preparation Methods
Synthetic Routes and Reaction Conditions: The synthesis of tubulysin M involves multiple steps, starting with the preparation of the individual amino acid components. These components are then coupled using peptide synthesis techniques. The key steps include the formation of N-methyl-D-pipecolic acid, L-isoleucine, tubuvaline, and tubuphenylalanine residues . The final coupling step involves the formation of the tetrapeptide backbone, followed by deprotection and purification.
Industrial Production Methods: Industrial production of this compound is challenging due to its complex structure and the difficulty in obtaining large quantities from natural sources. Advances in synthetic chemistry have enabled the production of this compound and its analogs through total synthesis . Metabolic engineering of myxobacteria to enhance the production of tubulysins is also being explored .
Chemical Reactions Analysis
Types of Reactions: Tubulysin M undergoes various chemical reactions, including:
Oxidation: this compound can be oxidized under specific conditions to form oxidized derivatives.
Reduction: Reduction reactions can modify the functional groups within this compound.
Substitution: Substitution reactions can introduce different functional groups into the this compound structure.
Common Reagents and Conditions:
Oxidation: Common oxidizing agents include hydrogen peroxide and potassium permanganate.
Reduction: Reducing agents such as sodium borohydride and lithium aluminum hydride are used.
Substitution: Reagents like alkyl halides and acyl chlorides are employed in substitution reactions.
Major Products: The major products formed from these reactions include various this compound derivatives with modified functional groups, which can exhibit different biological activities .
Scientific Research Applications
Tubulysin M has a wide range of scientific research applications, including:
Chemistry: this compound is used as a model compound for studying peptide synthesis and structure-activity relationships.
Biology: It is employed in cell biology research to study microtubule dynamics and cell division.
Mechanism of Action
Tubulysin M exerts its effects by binding to tubulin, a protein that is essential for microtubule formation. By inhibiting tubulin polymerization, this compound disrupts microtubule dynamics, leading to the collapse of the cytoskeleton and ultimately inducing apoptotic cell death . This mechanism is crucial for its effectiveness in targeting rapidly dividing cancer cells while sparing normal, healthy tissue .
Comparison with Similar Compounds
Structural and Functional Comparison with Other Tubulysins
Tubulysin A (TubA) and Pretubulysin (Prt)
- Potency: TubA and Prt exhibit comparable anti-angiogenic and tubulin polymerization inhibition activities (EC50 in the low nanomolar range). However, Tubulysin M is ~10-fold more potent than TubA in cytotoxicity assays .
- Stability : Prt, a biosynthetic precursor, lacks the C11 acetate but retains significant activity. Structural modifications, such as methylation or desmethylation, reduce potency by up to 100-fold, highlighting the importance of the C11 functional group .
- In Vivo Efficacy : Prt reduces tumor vascular density by 70% and completely inhibits tumor growth in murine models, demonstrating efficacy comparable to TubA but lower than this compound-based ADCs .
Tubulysin U and V
- Potency : Tubulysin U (IC50: 0.00065 nM in MDA-MB-435 cells) surpasses this compound in cytotoxicity, while Tubulysin V (IC50: 0.12 nM ) is less potent. Epimerization (e.g., epi-tubulysin V) reduces activity by 40-fold, emphasizing stereochemical sensitivity .
- Structural Differences : Tubulysin U and V feature variations in the N-terminal acyl group and methyl substituents, which influence tubulin binding affinity .
Comparison with Non-Tubulysin Microtubule Inhibitors
Monomethyl Auristatin E (MMAE)
- Potency : MMAE (IC50: 0.1–1 nM ) is less potent than this compound.
- Mechanism : Binds both α- and β-tubulin subunits, unlike this compound, which selectively targets the β-subunit. This difference enables this compound to overcome multidrug resistance (MDR) in Pgp-overexpressing cells .
- ADC Performance : this compound ADCs (e.g., anti-CD22 ADC 5) retain activity in MDR+ cell lines (IC50: 0.8 nM ), whereas MMAE ADCs lose efficacy entirely .
HTI-286 (Taltobulin)
- Structural Similarities : Shares a peptidic backbone but lacks the C11 acetate.
- Potency : HTI-286 (IC50: 0.00015 nM ) is slightly more potent than this compound but has lower plasma stability due to the absence of stabilizing substituents .
Key Structure-Activity Relationships (SAR)
- C11 Acetate : Removal reduces cytotoxicity by >100-fold (e.g., deacetylated this compound IC50: 2.4 nM ) .
- Tetrapeptide Backbone : Modifications (e.g., substitution of tubuvaline) disrupt hydrophobic interactions with tubulin pockets, reducing potency .
- Linker Chemistry : β-Glucuronidase-cleavable linkers enhance ADC stability and in vivo efficacy (e.g., 5/5 cure rate in L540cy models vs. 2/5 with dipeptide linkers) .
Data Tables
Table 1: Cytotoxicity of Tubulysins and Analogs
Table 2: ADC Performance in Preclinical Models
Biological Activity
Tubulysin M is a member of the tubulysin family, which are naturally occurring tetrapeptides produced by myxobacteria. These compounds exhibit significant cytotoxic activity by disrupting microtubule dynamics, leading to cell cycle arrest and apoptosis in cancer cells. Due to their potent anti-proliferative properties, tubulysins, including this compound, are being explored as potential candidates for antibody-drug conjugates (ADCs) in targeted cancer therapies.
This compound functions primarily through the inhibition of microtubule assembly. It binds to the vinca binding site on tubulin, effectively preventing the polymerization of tubulin into microtubules. This action results in the collapse of the cytoskeleton and triggers apoptotic pathways in cancer cells. The structural components of this compound include residues such as N-methyl-d-pipecolic acid, isoleucine, tubuvaline, and tubuphenylalanine, which are crucial for its biological activity.
Structure-Activity Relationship (SAR)
Research has focused on understanding how modifications to the structure of this compound can influence its biological activity. For instance, studies have shown that changes at the C-11 position can significantly affect both the affinity for tubulin and cytotoxicity against multidrug-resistant (MDR) cancer cell lines. The retention of a hydrolytically stable acetate group at C-11 is critical for maintaining potency in plasma environments .
In Vitro Activity
This compound has demonstrated exceptional potency against various cancer cell lines, including breast, colon, lung, ovarian, and prostate cancers. Its efficacy is particularly notable in MDR cell lines where conventional chemotherapeutics fail. For example, a study reported that this compound exhibited an EC50 in the picomolar range against several sensitive and resistant cancer cell lines .
In Vivo Efficacy
Despite its strong in vitro activity, this compound faces challenges in vivo due to systemic toxicity. Preclinical evaluations have indicated that while it shows promise as an ADC payload, its therapeutic window is narrow. For instance, conjugates of tubulysin with antibodies have been shown to retain significant activity against MDR tumors while minimizing systemic exposure . A notable case study involved a folate-tubulysin conjugate (EC1456), which demonstrated efficacy against subcutaneous tumors while combining with standard care agents .
Case Studies
- Stabilization Strategies : Research has focused on stabilizing tubulysin ADCs to enhance their therapeutic index. A study demonstrated that using a quaternary ammonium salt linker improved the stability and efficacy of tubulysin conjugates against lymphoma models resistant to traditional therapies .
- Glucuronide Linker Systems : The use of glucuronide linkers has shown promise in enhancing the stability and release profiles of tubulysin-based ADCs. These linkers exploit intracellular β-glucuronidase for drug release, demonstrating effective cytotoxicity across various MDR+ tumor models .
Comparative Data Table
Compound | Type | EC50 (nM) | Activity Against MDR | Stability |
---|---|---|---|---|
This compound | Natural Compound | <1 | Yes | Moderate |
EC1456 (Folate-Tub) | ADC | 0.5 | Yes | High |
MMAE (Control) | ADC | 1 | No | High |
Q & A
Basic Research Questions
Q. What analytical methods are recommended for quantifying Tubulysin M in complex biological matrices?
this compound quantification requires high sensitivity due to its low therapeutic index. Researchers should employ liquid chromatography-tandem mass spectrometry (LC-MS/MS) with isotope-labeled internal standards to minimize matrix effects. Method validation should follow FDA/ICH guidelines, including linearity (1–1000 ng/mL), precision (CV <15%), and recovery rates (>80%) . Ensure chromatographic separation optimizes peak symmetry to avoid co-eluting impurities, which can skew results .
Q. How can the microtubule-disrupting mechanism of this compound be experimentally validated?
Use immunofluorescence microscopy to visualize microtubule depolymerization in treated cancer cells (e.g., HeLa or A549 lines). Pair this with quantitative assays like tubulin polymerization inhibition (e.g., in vitro turbidity assays at 350 nm). Normalize data against controls (e.g., vinca alkaloids) and validate via Western blot for α-tubulin degradation . Ensure experimental replicates (n ≥ 3) to account for cell-line variability .
Q. What synthesis strategies are most effective for producing this compound analogs with improved stability?
Focus on modifying the labile N-terminal acyloxy moiety. Semi-synthetic approaches using solid-phase peptide synthesis (SPPS) for the tetrapeptide backbone, followed by late-stage functionalization of the Tubuvaline unit, can enhance metabolic stability. Validate purity via HPLC (>95%) and confirm stereochemistry via NMR (e.g., NOESY for spatial assignments) . Compare pharmacokinetic profiles (e.g., t½ in murine models) to assess improvements .
Advanced Research Questions
Q. How can researchers address discrepancies in this compound’s reported IC50 values across different cancer cell lines?
Discrepancies often arise from variations in assay conditions (e.g., serum concentration, incubation time). Standardize protocols using CLSI guidelines:
- Use identical passage-number cells.
- Pre-equilibrate culture media to 37°C/5% CO₂.
- Include a reference compound (e.g., paclitaxel) as an internal control. Apply multivariate regression to identify confounding variables (e.g., ATP levels in viability assays) .
Q. What experimental models are suitable for studying this compound resistance mechanisms?
Develop resistant cell lines via gradual dose escalation (e.g., over 6 months) and perform whole-exome sequencing to identify mutations in β-tubulin isoforms (e.g., TUBB3). Complement with CRISPR-Cas9 knock-in models to confirm resistance loci. Use patient-derived xenografts (PDXs) to validate findings in vivo and assess cross-resistance with other microtubule inhibitors .
Q. How can combination therapies involving this compound be optimized to mitigate systemic toxicity?
Design studies using factorial experimental designs to evaluate synergies (e.g., with immune checkpoint inhibitors). Prioritize agents that downregulate multidrug resistance (MDR) transporters (e.g., elacridar for P-gp inhibition). Use pharmacokinetic/pharmacodynamic (PK/PD) modeling to identify dosing schedules that maximize tumor exposure while minimizing hematological toxicity .
Q. What strategies improve the bioavailability of this compound in in vivo models?
Encapsulate this compound in lipid-based nanoparticles (LNPs) or PEGylated liposomes to enhance plasma half-life. Characterize formulations via dynamic light scattering (DLS) for size (50–100 nm) and zeta potential (±30 mV). Validate tumor targeting using near-infrared (NIR) imaging in orthotopic models .
Q. Methodological Considerations for Data Integrity
Q. How should researchers handle contradictory data in this compound’s dose-response relationships?
Apply sensitivity analysis to identify outlier datasets. Use tools like Grubbs’ test for statistical outliers and re-examine raw data (e.g., flow cytometry gating strategies). Transparently report all data, including negative results, in supplementary materials to avoid publication bias .
Q. What statistical frameworks are optimal for analyzing this compound’s synergistic effects in combination therapies?
Use the Chou-Talalay combination index (CI) method, where CI <1 indicates synergy. Pair this with Bliss independence models to account for non-interacting mechanisms. Validate with bootstrapping (1000 iterations) to estimate confidence intervals .
Q. Ethical and Reproducibility Guidelines
Q. How can researchers ensure reproducibility in this compound studies?
Adhere to ARRIVE 2.0 guidelines for in vivo experiments, including detailed reporting of animal husbandry and randomization. Share raw data (e.g., RNA-seq files) via repositories like GEO or Zenodo. Publish step-by-step synthetic protocols in supplementary materials, including NMR spectra and HPLC chromatograms .
Properties
IUPAC Name |
(2S,4R)-4-[[2-[(1R,3R)-1-acetyloxy-4-methyl-3-[methyl-[(2S,3S)-3-methyl-2-[[(2R)-1-methylpiperidine-2-carbonyl]amino]pentanoyl]amino]pentyl]-1,3-thiazole-4-carbonyl]amino]-2-methyl-5-phenylpentanoic acid | |
---|---|---|
Source | PubChem | |
URL | https://pubchem.ncbi.nlm.nih.gov | |
Description | Data deposited in or computed by PubChem | |
InChI |
InChI=1S/C38H57N5O7S/c1-9-24(4)33(41-35(46)30-17-13-14-18-42(30)7)37(47)43(8)31(23(2)3)21-32(50-26(6)44)36-40-29(22-51-36)34(45)39-28(19-25(5)38(48)49)20-27-15-11-10-12-16-27/h10-12,15-16,22-25,28,30-33H,9,13-14,17-21H2,1-8H3,(H,39,45)(H,41,46)(H,48,49)/t24-,25-,28+,30+,31+,32+,33-/m0/s1 | |
Source | PubChem | |
URL | https://pubchem.ncbi.nlm.nih.gov | |
Description | Data deposited in or computed by PubChem | |
InChI Key |
POBZYODNVHQLFG-ZRBKHQLFSA-N | |
Source | PubChem | |
URL | https://pubchem.ncbi.nlm.nih.gov | |
Description | Data deposited in or computed by PubChem | |
Canonical SMILES |
CCC(C)C(C(=O)N(C)C(CC(C1=NC(=CS1)C(=O)NC(CC2=CC=CC=C2)CC(C)C(=O)O)OC(=O)C)C(C)C)NC(=O)C3CCCCN3C | |
Source | PubChem | |
URL | https://pubchem.ncbi.nlm.nih.gov | |
Description | Data deposited in or computed by PubChem | |
Isomeric SMILES |
CC[C@H](C)[C@@H](C(=O)N(C)[C@H](C[C@H](C1=NC(=CS1)C(=O)N[C@@H](CC2=CC=CC=C2)C[C@H](C)C(=O)O)OC(=O)C)C(C)C)NC(=O)[C@H]3CCCCN3C | |
Source | PubChem | |
URL | https://pubchem.ncbi.nlm.nih.gov | |
Description | Data deposited in or computed by PubChem | |
Molecular Formula |
C38H57N5O7S | |
Source | PubChem | |
URL | https://pubchem.ncbi.nlm.nih.gov | |
Description | Data deposited in or computed by PubChem | |
Molecular Weight |
728.0 g/mol | |
Source | PubChem | |
URL | https://pubchem.ncbi.nlm.nih.gov | |
Description | Data deposited in or computed by PubChem | |
Retrosynthesis Analysis
AI-Powered Synthesis Planning: Our tool employs the Template_relevance Pistachio, Template_relevance Bkms_metabolic, Template_relevance Pistachio_ringbreaker, Template_relevance Reaxys, Template_relevance Reaxys_biocatalysis model, leveraging a vast database of chemical reactions to predict feasible synthetic routes.
One-Step Synthesis Focus: Specifically designed for one-step synthesis, it provides concise and direct routes for your target compounds, streamlining the synthesis process.
Accurate Predictions: Utilizing the extensive PISTACHIO, BKMS_METABOLIC, PISTACHIO_RINGBREAKER, REAXYS, REAXYS_BIOCATALYSIS database, our tool offers high-accuracy predictions, reflecting the latest in chemical research and data.
Strategy Settings
Precursor scoring | Relevance Heuristic |
---|---|
Min. plausibility | 0.01 |
Model | Template_relevance |
Template Set | Pistachio/Bkms_metabolic/Pistachio_ringbreaker/Reaxys/Reaxys_biocatalysis |
Top-N result to add to graph | 6 |
Feasible Synthetic Routes
Disclaimer and Information on In-Vitro Research Products
Please be aware that all articles and product information presented on BenchChem are intended solely for informational purposes. The products available for purchase on BenchChem are specifically designed for in-vitro studies, which are conducted outside of living organisms. In-vitro studies, derived from the Latin term "in glass," involve experiments performed in controlled laboratory settings using cells or tissues. It is important to note that these products are not categorized as medicines or drugs, and they have not received approval from the FDA for the prevention, treatment, or cure of any medical condition, ailment, or disease. We must emphasize that any form of bodily introduction of these products into humans or animals is strictly prohibited by law. It is essential to adhere to these guidelines to ensure compliance with legal and ethical standards in research and experimentation.