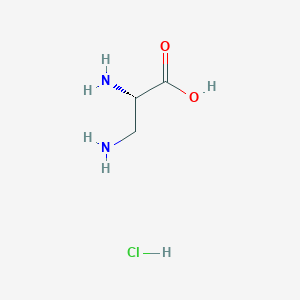
3-Amino-L-alanine hydrochloride
Overview
Description
3-Amino-L-alanine hydrochloride: is a non-proteinogenic amino acid with the molecular formula C3H9ClN2O2 and a molecular weight of 140.57 g/mol . It is commonly used in the synthesis of peptides containing non-proteinogenic amino acids . This compound appears as an off-white, hygroscopic powder and is slightly soluble in water and aqueous acids .
Mechanism of Action
Mode of Action
It is known to belong to the class of organic compounds known as L-alpha-amino acids . These are alpha amino acids which have the L-configuration of the alpha-carbon atom .
Biochemical Pathways
It is known that l-alpha-amino acids, the class of compounds to which 3-amino-l-alanine hydrochloride belongs, play crucial roles in various biochemical pathways, including protein synthesis .
Biochemical Analysis
Cellular Effects
It is believed to influence cell function, potentially impacting cell signaling pathways, gene expression, and cellular metabolism .
Molecular Mechanism
It is postulated that it may exert its effects at the molecular level through binding interactions with biomolecules, enzyme inhibition or activation, and changes in gene expression .
Temporal Effects in Laboratory Settings
The temporal effects of 3-Amino-L-alanine hydrochloride in laboratory settings, including its stability, degradation, and long-term effects on cellular function, are yet to be thoroughly investigated .
Dosage Effects in Animal Models
The effects of this compound at different dosages in animal models have not been extensively studied
Metabolic Pathways
The metabolic pathways that this compound is involved in, including any enzymes or cofactors it interacts with, are not well-defined
Preparation Methods
Synthetic Routes and Reaction Conditions: 3-Amino-L-alanine hydrochloride can be synthesized through the amidomalonate synthesis, which involves the conversion of diethyl acetamidomalonate into an enolate ion by treatment with a base, followed by S_N2 alkylation with a primary alkyl halide . The compound can be recrystallized from aqueous ethanol to obtain pure needles .
Industrial Production Methods: Industrial production of this compound typically involves large-scale synthesis using similar methods as described above, with optimization for yield and purity. The process may include additional purification steps such as recrystallization and chromatography to ensure the final product meets industrial standards .
Chemical Reactions Analysis
Types of Reactions: 3-Amino-L-alanine hydrochloride undergoes various chemical reactions, including:
Oxidation: The amino group can be oxidized to form corresponding oxo derivatives.
Reduction: The carboxyl group can be reduced to form alcohol derivatives.
Substitution: The amino group can participate in substitution reactions with various electrophiles
Common Reagents and Conditions:
Oxidation: Common oxidizing agents include potassium permanganate and hydrogen peroxide.
Reduction: Reducing agents such as lithium aluminum hydride and sodium borohydride are used.
Substitution: Electrophiles like acyl chlorides and alkyl halides are commonly used
Major Products Formed:
Oxidation: Formation of oxo derivatives.
Reduction: Formation of alcohol derivatives.
Substitution: Formation of substituted amino acids
Scientific Research Applications
3-Amino-L-alanine hydrochloride has a wide range of applications in scientific research:
Chemistry: Used in the synthesis of peptides containing non-proteinogenic amino acids.
Biology: Studied for its role in metabolic pathways and enzyme interactions.
Medicine: Investigated for its potential therapeutic effects and as a building block for drug synthesis.
Industry: Utilized in the production of fine chemicals and as a precursor for various biochemical compounds.
Comparison with Similar Compounds
L-alanine: A proteinogenic amino acid with similar structure but different biological roles.
3-Azido-L-alanine hydrochloride: An unnatural amino acid used for protein functionalization.
2,3-Diaminopropanoic acid hydrochloride: Another non-proteinogenic amino acid with similar properties.
Uniqueness: 3-Amino-L-alanine hydrochloride is unique due to its specific structure, which allows it to be used in the synthesis of peptides containing non-proteinogenic amino acids. This makes it valuable in research and industrial applications where such peptides are required .
Properties
IUPAC Name |
(2S)-2,3-diaminopropanoic acid;hydrochloride | |
---|---|---|
Source | PubChem | |
URL | https://pubchem.ncbi.nlm.nih.gov | |
Description | Data deposited in or computed by PubChem | |
InChI |
InChI=1S/C3H8N2O2.ClH/c4-1-2(5)3(6)7;/h2H,1,4-5H2,(H,6,7);1H/t2-;/m0./s1 | |
Source | PubChem | |
URL | https://pubchem.ncbi.nlm.nih.gov | |
Description | Data deposited in or computed by PubChem | |
InChI Key |
SKWCZPYWFRTSDD-DKWTVANSSA-N | |
Source | PubChem | |
URL | https://pubchem.ncbi.nlm.nih.gov | |
Description | Data deposited in or computed by PubChem | |
Canonical SMILES |
C(C(C(=O)O)N)N.Cl | |
Source | PubChem | |
URL | https://pubchem.ncbi.nlm.nih.gov | |
Description | Data deposited in or computed by PubChem | |
Isomeric SMILES |
C([C@@H](C(=O)O)N)N.Cl | |
Source | PubChem | |
URL | https://pubchem.ncbi.nlm.nih.gov | |
Description | Data deposited in or computed by PubChem | |
Molecular Formula |
C3H9ClN2O2 | |
Source | PubChem | |
URL | https://pubchem.ncbi.nlm.nih.gov | |
Description | Data deposited in or computed by PubChem | |
Molecular Weight |
140.57 g/mol | |
Source | PubChem | |
URL | https://pubchem.ncbi.nlm.nih.gov | |
Description | Data deposited in or computed by PubChem | |
CAS No. |
1482-97-9 | |
Record name | 2,3-Diaminopropionic acid hydrochloride, (+)- | |
Source | ChemIDplus | |
URL | https://pubchem.ncbi.nlm.nih.gov/substance/?source=chemidplus&sourceid=0001482979 | |
Description | ChemIDplus is a free, web search system that provides access to the structure and nomenclature authority files used for the identification of chemical substances cited in National Library of Medicine (NLM) databases, including the TOXNET system. | |
Record name | (S)-(+)-2,3-Diaminopropionic Acid Hydrochloride | |
Source | European Chemicals Agency (ECHA) | |
URL | https://echa.europa.eu/information-on-chemicals | |
Description | The European Chemicals Agency (ECHA) is an agency of the European Union which is the driving force among regulatory authorities in implementing the EU's groundbreaking chemicals legislation for the benefit of human health and the environment as well as for innovation and competitiveness. | |
Explanation | Use of the information, documents and data from the ECHA website is subject to the terms and conditions of this Legal Notice, and subject to other binding limitations provided for under applicable law, the information, documents and data made available on the ECHA website may be reproduced, distributed and/or used, totally or in part, for non-commercial purposes provided that ECHA is acknowledged as the source: "Source: European Chemicals Agency, http://echa.europa.eu/". Such acknowledgement must be included in each copy of the material. ECHA permits and encourages organisations and individuals to create links to the ECHA website under the following cumulative conditions: Links can only be made to webpages that provide a link to the Legal Notice page. | |
Record name | 2,3-DIAMINOPROPIONIC ACID HYDROCHLORIDE, (+)- | |
Source | FDA Global Substance Registration System (GSRS) | |
URL | https://gsrs.ncats.nih.gov/ginas/app/beta/substances/68H9573890 | |
Description | The FDA Global Substance Registration System (GSRS) enables the efficient and accurate exchange of information on what substances are in regulated products. Instead of relying on names, which vary across regulatory domains, countries, and regions, the GSRS knowledge base makes it possible for substances to be defined by standardized, scientific descriptions. | |
Explanation | Unless otherwise noted, the contents of the FDA website (www.fda.gov), both text and graphics, are not copyrighted. They are in the public domain and may be republished, reprinted and otherwise used freely by anyone without the need to obtain permission from FDA. Credit to the U.S. Food and Drug Administration as the source is appreciated but not required. | |
Retrosynthesis Analysis
AI-Powered Synthesis Planning: Our tool employs the Template_relevance Pistachio, Template_relevance Bkms_metabolic, Template_relevance Pistachio_ringbreaker, Template_relevance Reaxys, Template_relevance Reaxys_biocatalysis model, leveraging a vast database of chemical reactions to predict feasible synthetic routes.
One-Step Synthesis Focus: Specifically designed for one-step synthesis, it provides concise and direct routes for your target compounds, streamlining the synthesis process.
Accurate Predictions: Utilizing the extensive PISTACHIO, BKMS_METABOLIC, PISTACHIO_RINGBREAKER, REAXYS, REAXYS_BIOCATALYSIS database, our tool offers high-accuracy predictions, reflecting the latest in chemical research and data.
Strategy Settings
Precursor scoring | Relevance Heuristic |
---|---|
Min. plausibility | 0.01 |
Model | Template_relevance |
Template Set | Pistachio/Bkms_metabolic/Pistachio_ringbreaker/Reaxys/Reaxys_biocatalysis |
Top-N result to add to graph | 6 |
Feasible Synthetic Routes
Disclaimer and Information on In-Vitro Research Products
Please be aware that all articles and product information presented on BenchChem are intended solely for informational purposes. The products available for purchase on BenchChem are specifically designed for in-vitro studies, which are conducted outside of living organisms. In-vitro studies, derived from the Latin term "in glass," involve experiments performed in controlled laboratory settings using cells or tissues. It is important to note that these products are not categorized as medicines or drugs, and they have not received approval from the FDA for the prevention, treatment, or cure of any medical condition, ailment, or disease. We must emphasize that any form of bodily introduction of these products into humans or animals is strictly prohibited by law. It is essential to adhere to these guidelines to ensure compliance with legal and ethical standards in research and experimentation.