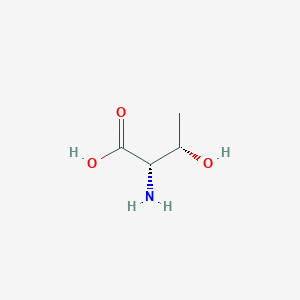
L-allo-threonine
Overview
Description
H-allo-Thr-OH: , also known as L-Allothreonine, is an endogenous metabolite. It is a stereoisomer of threonine, specifically the allo form, which means it has a different spatial arrangement of atoms compared to the common threonine. The compound has the molecular formula C4H9NO3 and a molecular weight of 119.12 g/mol . It is a white to off-white solid and is classified under ketones, aldehydes, and acids .
Mechanism of Action
Target of Action
L-Allothreonine, also known as L-allo-threonine, primarily targets Threonine Aldolases (TAs) . TAs are a class of enzymes that play a crucial role in catalyzing carbon-carbon bond formations in synthetic organic chemistry . They are pyridoxal-5-phosphate-dependent enzymes, which, in nature, catalyze the cleavage of L-threonine or this compound to glycine and acetaldehyde in a glycine biosynthetic pathway .
Mode of Action
L-Allothreonine interacts with its target, Threonine Aldolases, to catalyze the formation of β-hydroxy-α-amino acids . This process involves the conversion of the achiral precursors glycine and an aldehyde into two new stereogenic centers . The interaction results in the enantio- and diastereoselective synthesis of β-hydroxy-α-amino acids .
Biochemical Pathways
The action of L-Allothreonine affects the glycine biosynthetic pathway . In this pathway, Threonine Aldolases catalyze the cleavage of L-threonine or this compound to glycine and acetaldehyde . The resulting chiral β-hydroxy-α-amino acid products are important precursors for various pharmaceuticals .
Pharmacokinetics
It’s worth noting that l-allothreonine is a water-soluble colorless solid , which may influence its bioavailability and distribution in the body.
Result of Action
The action of L-Allothreonine results in the production of β-hydroxy-α-amino acids . These compounds are valuable in synthetic organic chemistry due to their stereocontrolled formation . They serve as important precursors for various pharmaceuticals .
Action Environment
The action of L-Allothreonine can be influenced by various environmental factors. For instance, L-Allothreonine is a plant metabolite that appears in the human diet in variable concentrations depending on plant species, physiological changes during plant growth, senescence, and reactions to environmental stress or changes due to plant transformation . Additionally, this compound aldolase, the enzyme that L-Allothreonine targets, is a highly thermostable enzyme, which means it can survive at temperatures up to 60 degrees Celsius . This thermostability can be used for purification and may influence the compound’s action, efficacy, and stability .
Biochemical Analysis
Biochemical Properties
L-Allothreonine is involved in biochemical reactions catalyzed by threonine aldolases (TAs), which are pyridoxal-5-phosphate-dependent enzymes . These enzymes catalyze the reversible cleavage of L-allothreonine to glycine and acetaldehyde, a critical step in the glycine biosynthetic pathway . The interaction between L-Allothreonine and TAs forms two new stereogenic centers, enabling an enantio- and diastereoselective synthesis of β-hydroxy-α-amino acids .
Cellular Effects
The conversion of L-Allothreonine to glycine and acetaldehyde by TAs has significant implications for cellular function. This process contributes to the production of glycine, an essential amino acid for protein synthesis . Additionally, the acetaldehyde produced can enter various metabolic pathways, influencing cellular metabolism .
Molecular Mechanism
The molecular mechanism of L-Allothreonine involves its interaction with TAs. These enzymes, dependent on pyridoxal-5-phosphate, catalyze the cleavage of L-Allothreonine to produce glycine and acetaldehyde . This reaction involves the formation of carbon–carbon bonds, leading to the synthesis of β-hydroxy-α-amino acids .
Metabolic Pathways
L-Allothreonine is involved in the glycine biosynthetic pathway, where it is cleaved by TAs to produce glycine and acetaldehyde . This process is a key part of the metabolism of L-Allothreonine.
Preparation Methods
Synthetic Routes and Reaction Conditions: L-Allothreonine can be synthesized through various methods. One common approach involves the use of threonine aldolases, which are enzymes that catalyze the formation of carbon-carbon bonds. These enzymes enable the enantio- and diastereoselective synthesis of β-hydroxy-α-amino acids starting from achiral precursors like glycine and an aldehyde . The reaction conditions typically involve mild temperatures and neutral pH to maintain enzyme activity.
Industrial Production Methods: In industrial settings, the production of L-Allothreonine often involves microbial fermentation processes. Specific strains of bacteria or fungi are engineered to overproduce the enzyme threonine aldolase, which then catalyzes the formation of L-Allothreonine from simpler substrates. The product is then purified through crystallization or other separation techniques .
Chemical Reactions Analysis
Types of Reactions: L-Allothreonine undergoes various chemical reactions, including:
Oxidation: It can be oxidized to form corresponding keto acids.
Reduction: Reduction reactions can convert it into different amino alcohols.
Substitution: It can participate in substitution reactions where the hydroxyl group is replaced by other functional groups.
Common Reagents and Conditions:
Oxidation: Common oxidizing agents include potassium permanganate and chromium trioxide.
Reduction: Reducing agents like sodium borohydride or lithium aluminum hydride are often used.
Substitution: Conditions vary depending on the desired product, but common reagents include halogenating agents and nucleophiles.
Major Products:
Oxidation: Keto acids.
Reduction: Amino alcohols.
Substitution: Various substituted threonine derivatives.
Scientific Research Applications
L-Allothreonine has several applications in scientific research:
Comparison with Similar Compounds
L-Threonine: The most common isomer of threonine, differing in the spatial arrangement of atoms.
D-Allothreonine: The D-isomer of allothreonine, which has different optical activity.
L-Serine: Another amino acid with a similar structure but lacking the methyl group present in threonine.
Uniqueness: L-Allothreonine is unique due to its specific stereochemistry, which imparts distinct biochemical properties. Its ability to participate in specific enzymatic reactions makes it valuable for research and industrial applications .
Properties
IUPAC Name |
(2S,3S)-2-amino-3-hydroxybutanoic acid | |
---|---|---|
Source | PubChem | |
URL | https://pubchem.ncbi.nlm.nih.gov | |
Description | Data deposited in or computed by PubChem | |
InChI |
InChI=1S/C4H9NO3/c1-2(6)3(5)4(7)8/h2-3,6H,5H2,1H3,(H,7,8)/t2-,3-/m0/s1 | |
Source | PubChem | |
URL | https://pubchem.ncbi.nlm.nih.gov | |
Description | Data deposited in or computed by PubChem | |
InChI Key |
AYFVYJQAPQTCCC-HRFVKAFMSA-N | |
Source | PubChem | |
URL | https://pubchem.ncbi.nlm.nih.gov | |
Description | Data deposited in or computed by PubChem | |
Canonical SMILES |
CC(C(C(=O)O)N)O | |
Source | PubChem | |
URL | https://pubchem.ncbi.nlm.nih.gov | |
Description | Data deposited in or computed by PubChem | |
Isomeric SMILES |
C[C@@H]([C@@H](C(=O)O)N)O | |
Source | PubChem | |
URL | https://pubchem.ncbi.nlm.nih.gov | |
Description | Data deposited in or computed by PubChem | |
Molecular Formula |
C4H9NO3 | |
Source | PubChem | |
URL | https://pubchem.ncbi.nlm.nih.gov | |
Description | Data deposited in or computed by PubChem | |
DSSTOX Substance ID |
DTXSID10183151, DTXSID301030659 | |
Record name | Allo-L-threonine | |
Source | EPA DSSTox | |
URL | https://comptox.epa.gov/dashboard/DTXSID10183151 | |
Description | DSSTox provides a high quality public chemistry resource for supporting improved predictive toxicology. | |
Record name | Allothreonine | |
Source | EPA DSSTox | |
URL | https://comptox.epa.gov/dashboard/DTXSID301030659 | |
Description | DSSTox provides a high quality public chemistry resource for supporting improved predictive toxicology. | |
Molecular Weight |
119.12 g/mol | |
Source | PubChem | |
URL | https://pubchem.ncbi.nlm.nih.gov | |
Description | Data deposited in or computed by PubChem | |
Physical Description |
Solid | |
Record name | L-Allothreonine | |
Source | Human Metabolome Database (HMDB) | |
URL | http://www.hmdb.ca/metabolites/HMDB0004041 | |
Description | The Human Metabolome Database (HMDB) is a freely available electronic database containing detailed information about small molecule metabolites found in the human body. | |
Explanation | HMDB is offered to the public as a freely available resource. Use and re-distribution of the data, in whole or in part, for commercial purposes requires explicit permission of the authors and explicit acknowledgment of the source material (HMDB) and the original publication (see the HMDB citing page). We ask that users who download significant portions of the database cite the HMDB paper in any resulting publications. | |
CAS No. |
144-98-9, 28954-12-3, 24830-94-2 | |
Record name | Allothreonine | |
Source | CAS Common Chemistry | |
URL | https://commonchemistry.cas.org/detail?cas_rn=144-98-9 | |
Description | CAS Common Chemistry is an open community resource for accessing chemical information. Nearly 500,000 chemical substances from CAS REGISTRY cover areas of community interest, including common and frequently regulated chemicals, and those relevant to high school and undergraduate chemistry classes. This chemical information, curated by our expert scientists, is provided in alignment with our mission as a division of the American Chemical Society. | |
Explanation | The data from CAS Common Chemistry is provided under a CC-BY-NC 4.0 license, unless otherwise stated. | |
Record name | L-Allothreonine | |
Source | CAS Common Chemistry | |
URL | https://commonchemistry.cas.org/detail?cas_rn=28954-12-3 | |
Description | CAS Common Chemistry is an open community resource for accessing chemical information. Nearly 500,000 chemical substances from CAS REGISTRY cover areas of community interest, including common and frequently regulated chemicals, and those relevant to high school and undergraduate chemistry classes. This chemical information, curated by our expert scientists, is provided in alignment with our mission as a division of the American Chemical Society. | |
Explanation | The data from CAS Common Chemistry is provided under a CC-BY-NC 4.0 license, unless otherwise stated. | |
Record name | Allothreonine | |
Source | ChemIDplus | |
URL | https://pubchem.ncbi.nlm.nih.gov/substance/?source=chemidplus&sourceid=0028954123 | |
Description | ChemIDplus is a free, web search system that provides access to the structure and nomenclature authority files used for the identification of chemical substances cited in National Library of Medicine (NLM) databases, including the TOXNET system. | |
Record name | Allo-L-threonine | |
Source | EPA DSSTox | |
URL | https://comptox.epa.gov/dashboard/DTXSID10183151 | |
Description | DSSTox provides a high quality public chemistry resource for supporting improved predictive toxicology. | |
Record name | Allothreonine | |
Source | EPA DSSTox | |
URL | https://comptox.epa.gov/dashboard/DTXSID301030659 | |
Description | DSSTox provides a high quality public chemistry resource for supporting improved predictive toxicology. | |
Record name | Allo-L-threonine | |
Source | European Chemicals Agency (ECHA) | |
URL | https://echa.europa.eu/substance-information/-/substanceinfo/100.044.829 | |
Description | The European Chemicals Agency (ECHA) is an agency of the European Union which is the driving force among regulatory authorities in implementing the EU's groundbreaking chemicals legislation for the benefit of human health and the environment as well as for innovation and competitiveness. | |
Explanation | Use of the information, documents and data from the ECHA website is subject to the terms and conditions of this Legal Notice, and subject to other binding limitations provided for under applicable law, the information, documents and data made available on the ECHA website may be reproduced, distributed and/or used, totally or in part, for non-commercial purposes provided that ECHA is acknowledged as the source: "Source: European Chemicals Agency, http://echa.europa.eu/". Such acknowledgement must be included in each copy of the material. ECHA permits and encourages organisations and individuals to create links to the ECHA website under the following cumulative conditions: Links can only be made to webpages that provide a link to the Legal Notice page. | |
Record name | Allo-DL-threonine | |
Source | European Chemicals Agency (ECHA) | |
URL | https://echa.europa.eu/substance-information/-/substanceinfo/100.005.133 | |
Description | The European Chemicals Agency (ECHA) is an agency of the European Union which is the driving force among regulatory authorities in implementing the EU's groundbreaking chemicals legislation for the benefit of human health and the environment as well as for innovation and competitiveness. | |
Explanation | Use of the information, documents and data from the ECHA website is subject to the terms and conditions of this Legal Notice, and subject to other binding limitations provided for under applicable law, the information, documents and data made available on the ECHA website may be reproduced, distributed and/or used, totally or in part, for non-commercial purposes provided that ECHA is acknowledged as the source: "Source: European Chemicals Agency, http://echa.europa.eu/". Such acknowledgement must be included in each copy of the material. ECHA permits and encourages organisations and individuals to create links to the ECHA website under the following cumulative conditions: Links can only be made to webpages that provide a link to the Legal Notice page. | |
Record name | ALLOTHREONINE, L- | |
Source | FDA Global Substance Registration System (GSRS) | |
URL | https://gsrs.ncats.nih.gov/ginas/app/beta/substances/HCQ253CKVK | |
Description | The FDA Global Substance Registration System (GSRS) enables the efficient and accurate exchange of information on what substances are in regulated products. Instead of relying on names, which vary across regulatory domains, countries, and regions, the GSRS knowledge base makes it possible for substances to be defined by standardized, scientific descriptions. | |
Explanation | Unless otherwise noted, the contents of the FDA website (www.fda.gov), both text and graphics, are not copyrighted. They are in the public domain and may be republished, reprinted and otherwise used freely by anyone without the need to obtain permission from FDA. Credit to the U.S. Food and Drug Administration as the source is appreciated but not required. | |
Record name | ALLOTHREONINE, DL- | |
Source | FDA Global Substance Registration System (GSRS) | |
URL | https://gsrs.ncats.nih.gov/ginas/app/beta/substances/29NAP6417F | |
Description | The FDA Global Substance Registration System (GSRS) enables the efficient and accurate exchange of information on what substances are in regulated products. Instead of relying on names, which vary across regulatory domains, countries, and regions, the GSRS knowledge base makes it possible for substances to be defined by standardized, scientific descriptions. | |
Explanation | Unless otherwise noted, the contents of the FDA website (www.fda.gov), both text and graphics, are not copyrighted. They are in the public domain and may be republished, reprinted and otherwise used freely by anyone without the need to obtain permission from FDA. Credit to the U.S. Food and Drug Administration as the source is appreciated but not required. | |
Record name | L-Allothreonine | |
Source | Human Metabolome Database (HMDB) | |
URL | http://www.hmdb.ca/metabolites/HMDB0004041 | |
Description | The Human Metabolome Database (HMDB) is a freely available electronic database containing detailed information about small molecule metabolites found in the human body. | |
Explanation | HMDB is offered to the public as a freely available resource. Use and re-distribution of the data, in whole or in part, for commercial purposes requires explicit permission of the authors and explicit acknowledgment of the source material (HMDB) and the original publication (see the HMDB citing page). We ask that users who download significant portions of the database cite the HMDB paper in any resulting publications. | |
Melting Point |
256 °C | |
Record name | L-Allothreonine | |
Source | Human Metabolome Database (HMDB) | |
URL | http://www.hmdb.ca/metabolites/HMDB0004041 | |
Description | The Human Metabolome Database (HMDB) is a freely available electronic database containing detailed information about small molecule metabolites found in the human body. | |
Explanation | HMDB is offered to the public as a freely available resource. Use and re-distribution of the data, in whole or in part, for commercial purposes requires explicit permission of the authors and explicit acknowledgment of the source material (HMDB) and the original publication (see the HMDB citing page). We ask that users who download significant portions of the database cite the HMDB paper in any resulting publications. | |
Retrosynthesis Analysis
AI-Powered Synthesis Planning: Our tool employs the Template_relevance Pistachio, Template_relevance Bkms_metabolic, Template_relevance Pistachio_ringbreaker, Template_relevance Reaxys, Template_relevance Reaxys_biocatalysis model, leveraging a vast database of chemical reactions to predict feasible synthetic routes.
One-Step Synthesis Focus: Specifically designed for one-step synthesis, it provides concise and direct routes for your target compounds, streamlining the synthesis process.
Accurate Predictions: Utilizing the extensive PISTACHIO, BKMS_METABOLIC, PISTACHIO_RINGBREAKER, REAXYS, REAXYS_BIOCATALYSIS database, our tool offers high-accuracy predictions, reflecting the latest in chemical research and data.
Strategy Settings
Precursor scoring | Relevance Heuristic |
---|---|
Min. plausibility | 0.01 |
Model | Template_relevance |
Template Set | Pistachio/Bkms_metabolic/Pistachio_ringbreaker/Reaxys/Reaxys_biocatalysis |
Top-N result to add to graph | 6 |
Feasible Synthetic Routes
Q1: What is L-allo-threonine and how is it different from L-threonine?
A1: this compound (L-aThr) is a stereoisomer of the proteinogenic amino acid L-threonine (L-Thr). Stereoisomers share the same molecular formula and connectivity but differ in the spatial arrangement of their atoms. In the case of L-aThr and L-Thr, they are diastereomers, meaning they are stereoisomers that are not mirror images of each other. This difference arises from the chirality at the beta-carbon atom, leading to distinct biological properties.
Q2: How does the stereochemistry of this compound impact its recognition by enzymes?
A2: The distinct stereochemistry of L-aThr plays a crucial role in its interaction with enzymes. Studies on L-threonine aldolase (LTA), a pyridoxal 5'-phosphate-dependent enzyme, highlight this aspect. While LTA from certain organisms shows activity towards both L-Thr and L-aThr, the enzyme exhibits a strong preference for L-aThr []. This selectivity stems from the specific interactions within the enzyme's active site, where subtle differences in the spatial arrangement of the hydroxyl and methyl groups on the beta-carbon of the substrate dictate binding affinity and catalytic efficiency.
Q3: Can you elaborate on the structural basis of substrate specificity in this compound aldolase?
A3: Crystallographic studies on this compound aldolase (LATA) from Aeromonas jandaei DK-39, an enzyme highly specific for L-aThr, provide insights into the structural basis of substrate recognition []. The enzyme's active site possesses a loop region (residues Ala123-Pro131) that undergoes conformational changes upon substrate binding. Specifically, a histidine residue (His128) within this loop plays a crucial role in discriminating between L-aThr and L-Thr. Mutations at this position, particularly the H128Y mutation, significantly alter the enzyme's stereoselectivity, highlighting the importance of this residue in substrate recognition [].
Q4: What is the role of pyridoxal 5'-phosphate (PLP) in this compound aldolase activity?
A4: this compound aldolase (LATA) requires PLP as a cofactor for its catalytic activity [, , ]. PLP, a derivative of vitamin B6, forms a Schiff base linkage with a lysine residue (Lys199) in the active site of LATA []. This Schiff base acts as an electron sink, facilitating the aldol cleavage reaction of L-aThr into glycine and acetaldehyde. This mechanism is supported by studies where mutations of the PLP-binding lysine residue completely abolish the enzyme's activity [].
Q5: What are the potential applications of this compound aldolase in biotechnology?
A5: this compound aldolase (LATA) holds potential for biotechnological applications, particularly in the enzymatic synthesis of L-aThr. Given its high stereoselectivity, LATA could be employed to produce enantiomerically pure L-aThr, a valuable chiral building block for pharmaceuticals and other fine chemicals. Additionally, engineered LATA variants with altered substrate specificity, such as those exhibiting enhanced activity towards L-Thr, could be utilized for the production of L-Thr, an essential amino acid [].
Q6: Beyond this compound aldolase, are there other enzymes that utilize this compound as a substrate?
A6: Yes, this compound can be utilized by other enzymes. For instance, yeast cystathionine β-synthase, a PLP-dependent enzyme, can react with L-aThr and L-homocysteine to form a novel amino acid, 3-methyl-L-cystathionine []. This finding demonstrates the potential of L-aThr as a substrate for enzymatic synthesis of non-natural amino acids, expanding its applications in biocatalysis.
Q7: Are there any studies on the formation of mixed crystals between L-threonine and this compound?
A7: Yes, research has explored the formation of mixed crystals between L-Thr and L-aThr. Notably, studies have shown that D- and L-threonine crystals can be contaminated with D- and L-allothreonine, respectively, suggesting the formation of mixed crystals during crystallization processes []. This finding is relevant for the separation and purification of these diastereomers, as their similar physicochemical properties pose challenges for their isolation.
Q8: What analytical techniques are employed to differentiate between L-threonine and this compound?
A8: Distinguishing L-Thr from L-aThr requires techniques capable of differentiating stereoisomers. Here are a few examples:
- Terahertz time-domain spectroscopy (THz-TDS): THz-TDS can differentiate between diastereomers based on their unique terahertz absorption spectra, influenced by differences in intermolecular interactions and vibrational modes [, ]. This technique has been successfully applied to discriminate between L-Thr, L-aThr, and their respective enantiomers [, ].
- Nuclear Magnetic Resonance (NMR) spectroscopy: NMR can distinguish diastereomers through characteristic differences in chemical shifts and coupling constants. Notably, the chemical shifts of the C-methyl groups in L-Thr and L-aThr differ significantly, allowing for their differentiation by NMR [].
Q9: Are there any computational studies on the conformational flexibility of this compound?
A9: Yes, computational studies employing density functional theory (DFT) have been conducted to explore the conformational flexibility of both L-Thr and L-aThr [, ]. These studies reveal a complex conformational landscape for both amino acids, with multiple stable conformers identified in the gas phase and aqueous solution. The relative stability of these conformers is influenced by intramolecular hydrogen bonding patterns, particularly those involving the hydroxyl group on the beta-carbon [, ].
Q10: Has this compound been found in any natural products?
A10: Yes, this compound is a constituent of certain natural products, notably peptide antibiotics. Globomycin, a cyclic peptide antibiotic known for its spheroplast-forming activity, contains this compound as one of its amino acid components []. Another example is the antibiotic LL-A-0341, which also incorporates this compound in its structure []. The presence of this compound in these biologically active molecules highlights its significance in natural product biosynthesis and its potential as a building block for novel therapeutics.
Q11: Can this compound be produced enzymatically at a larger scale?
A11: ** Research has explored the potential of using enzymatic methods for the preparative-scale production of this compound. One study investigated the diastereospecific formation of this compound from glycine and acetaldehyde using recombinant serine hydroxymethyltransferase (GlyA) from Escherichia coli []. The enzyme exhibited favorable stability and activity, even in the presence of organic cosolvents, suggesting its potential for use in continuous bioprocesses for this compound production. Further research is needed to optimize such biocatalytic processes for industrial-scale applications.
Q12: Are there any known applications of this compound in the synthesis of more complex molecules?
A12: ** Yes, this compound has been employed in the synthesis of complex molecules, particularly in carbohydrate chemistry. One example is the total synthesis of the tetrasaccharide repeating unit of Vibrio cholerae O43 [, ]. This complex carbohydrate contains this compound linked to a challenging to synthesize tetrasaccharide. This synthesis highlights the utility of this compound as a building block in the construction of complex oligosaccharides, which are valuable targets for the development of vaccines and other therapeutics.
Disclaimer and Information on In-Vitro Research Products
Please be aware that all articles and product information presented on BenchChem are intended solely for informational purposes. The products available for purchase on BenchChem are specifically designed for in-vitro studies, which are conducted outside of living organisms. In-vitro studies, derived from the Latin term "in glass," involve experiments performed in controlled laboratory settings using cells or tissues. It is important to note that these products are not categorized as medicines or drugs, and they have not received approval from the FDA for the prevention, treatment, or cure of any medical condition, ailment, or disease. We must emphasize that any form of bodily introduction of these products into humans or animals is strictly prohibited by law. It is essential to adhere to these guidelines to ensure compliance with legal and ethical standards in research and experimentation.