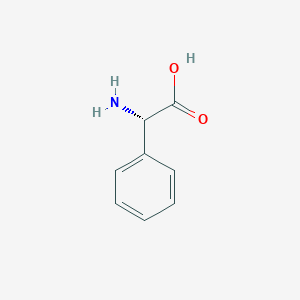
L-Phenylglycine
Overview
Description
L-Phenylglycine (C₈H₉NO₂) is a non-proteinogenic amino acid widely used as a chiral building block in pharmaceuticals, including β-lactam antibiotics (e.g., ampicillin), antitumor agents (e.g., taxol), and glycopeptide antibiotics like virginiamycin S . Traditional chemical synthesis methods rely on petrochemical feedstocks, which involve toxic reagents, low enantioselectivity, and environmental hazards . In contrast, microbial bioconversion offers a sustainable alternative with high enantiomeric excess (ee > 99%) and scalability, making it the preferred industrial method .
Mechanism of Action
- Peptide Natural Products : L-Phg is found in glycopeptide antibiotics and biologically active linear and cyclic peptides . These compounds play essential roles in various biological processes.
Mode of Action
The interaction of L-Phg with its targets involves several mechanisms:
- Biosynthesis : L-Phg is biosynthesized through specific pathways. For example, in Streptomyces pristinaespiralis, it originates from the shikimate pathway, where phenylpyruvate is converted to phenylacetyl-CoA via a pyruvate dehydrogenase-like complex . This biosynthesis contributes to the incorporation of L-Phg into peptide natural products.
Pharmacokinetics
The pharmacokinetic properties of L-Phg impact its bioavailability:
- Absorption : L-Phg can be absorbed through the intestinal peptide transporter I (PepT1) in the intestine. Strategies like using d-phenylglycine as a delivery tool have been explored to enhance L-Phg absorption .
Result of Action
The molecular and cellular effects of L-Phg are diverse:
- Drug Synthesis : L-Phg serves as a precursor for the production of semisynthetic β-lactam antibiotics, such as penicillin and virginiamycin S . Its incorporation into these drugs influences their bioactivity.
Action Environment
Environmental factors play a role in L-Phg’s efficacy and stability:
Biochemical Analysis
Biochemical Properties
L-Phenylglycine is involved in biochemical reactions through the co-expression of leucine dehydrogenase from Bacillus cereus (BcLeuDH) and NAD±dependent mutant formate dehydrogenase from Candida boidinii (CbFDHA10C) in Escherichia coli . These enzymes interact with this compound, facilitating its conversion from benzoylformic acid .
Cellular Effects
The effects of this compound on cells are primarily observed in its role in the synthesis of antibiotics and antitumor compounds . It influences cell function by participating in these synthesis processes, which can impact cell signaling pathways, gene expression, and cellular metabolism .
Molecular Mechanism
The molecular mechanism of this compound involves its conversion from benzoylformic acid through the action of BcLeuDH and CbFDHA10C . This process involves enzyme activation and changes in gene expression within the host organism .
Temporal Effects in Laboratory Settings
The effects of this compound over time in laboratory settings are observed in its role in the synthesis of antibiotics and antitumor compounds . Information on its stability, degradation, and long-term effects on cellular function is subject to the specific conditions of each experiment .
Dosage Effects in Animal Models
The effects of this compound at different dosages in animal models are subject to the specific conditions of each experiment . Its effects can vary depending on the dosage, with potential toxic or adverse effects at high doses .
Metabolic Pathways
This compound is involved in the metabolic pathways of antibiotic and antitumor compound synthesis . It interacts with enzymes and cofactors in these pathways, potentially affecting metabolic flux or metabolite levels .
Transport and Distribution
The transport and distribution of this compound within cells and tissues are facilitated by its interactions with enzymes and cofactors in its metabolic pathways . These interactions can affect its localization or accumulation within the cell .
Subcellular Localization
The subcellular localization of this compound is determined by its role in the metabolic pathways of antibiotic and antitumor compound synthesis . It may be directed to specific compartments or organelles based on the requirements of these pathways .
Biological Activity
L-Phenylglycine (L-Phg) is a non-proteinogenic amino acid that has garnered attention due to its diverse biological activities and applications in pharmaceuticals, particularly as a precursor for β-lactam antibiotics and anticancer agents. This article explores the biological activity of this compound, focusing on its synthesis, physiological effects, and potential therapeutic applications.
1. Synthesis of this compound
This compound can be synthesized through various enzymatic pathways. Recent advancements have demonstrated efficient production methods using engineered microorganisms. For instance, a study reported a four-step enzymatic cascade that converts L-phenylalanine to L-Phg, achieving significant yields through optimized fermentation conditions . The introduction of cofactor regeneration systems has also enhanced production efficiency, allowing for higher concentrations of L-Phg to be achieved in biocatalytic processes .
2.1 Appetite Regulation and Glycaemic Control
Research indicates that this compound influences appetite regulation and glycaemic response. A study evaluated the effects of intragastric administration of phenylalanine (the precursor of L-Phg) on appetite and energy intake. Results showed that higher doses of phenylalanine significantly stimulated the release of cholecystokinin (CCK), which is associated with appetite suppression, and improved glycaemic control by reducing plasma glucose levels . The findings suggest that L-Phg may play a role in metabolic regulation through its impact on gut hormones.
2.2 Inhibition of Key Enzymes
Novel derivatives of this compound have been synthesized and evaluated for their biological activity, particularly as potential inhibitors of key metabolic enzymes such as α-glucosidase and dipeptidyl peptidase-4 (DPP-4). One study reported that certain L-Phg derivatives exhibited significant inhibitory effects on these enzymes, which are crucial targets in the management of type 2 diabetes . The ability to modulate these enzymes highlights the therapeutic potential of L-Phg derivatives in metabolic disorders.
3.1 Antitumor Activity
This compound has been investigated for its antitumor properties as well. A case study explored its role in enhancing the efficacy of taxol, a well-known anticancer drug. The study found that L-Phg could potentiate the effects of taxol in cancer cell lines, suggesting a synergistic relationship that could be exploited in cancer therapy .
3.2 Allergic Reactions
Despite its beneficial properties, there are reports of hypersensitivity reactions associated with exposure to phenylglycine compounds. A clinical case documented respiratory allergies triggered by phenylglycine acid chloride, emphasizing the need for caution in its use . Understanding these adverse effects is crucial for developing safer therapeutic applications.
4. Comparative Analysis of Biological Activity
The following table summarizes key findings from various studies concerning the biological activity of this compound:
5. Conclusion
This compound exhibits a range of biological activities that make it a valuable compound in both research and therapeutic contexts. Its ability to regulate appetite, influence glycaemic control, and serve as a precursor for important pharmaceutical agents underscores its significance in biochemistry and medicine. Ongoing research into its derivatives may further enhance its therapeutic potential while addressing safety concerns associated with allergic reactions.
Scientific Research Applications
Pharmaceutical Applications
1.1 Antidiabetic Properties
Research has identified L-Phenylglycine derivatives as potential antidiabetic agents. A study detailed the design and synthesis of novel this compound derivatives, which exhibited significant hypoglycemic activity. These compounds were shown to act as agonists for peroxisome proliferator-activated receptors (PPARs), which are crucial in glucose metabolism and insulin sensitivity. Specifically, certain derivatives demonstrated agonist activity levels comparable to established medications like pioglitazone, suggesting their potential as low-toxicity antidiabetic drugs .
1.2 Inhibition of Amino Acid Transporters
This compound and its analogs have been studied for their inhibitory effects on neutral amino acid transporters ASCT1 and ASCT2. These transporters are involved in the uptake of d-serine, which is critical for NMDA receptor activity in the central nervous system. The inhibition of these transporters by this compound enhances long-term potentiation (LTP) in neuronal tissues, indicating its potential therapeutic benefits in neurodegenerative diseases .
Biochemical Research
2.1 Modulation of Amyloid Formation
In the context of phenylketonuria (PKU), this compound has been investigated for its ability to modulate amyloid formation associated with L-phenylalanine aggregation. D-Phenylglycine was found to inhibit the fibril formation of L-phenylalanine, suggesting a therapeutic role for D-phenylglycine in managing PKU by preventing toxic aggregation .
2.2 Enzymatic Synthesis
Recent advancements have demonstrated efficient enzymatic pathways for synthesizing this compound from biobased L-phenylalanine using a multi-enzyme cascade. This approach not only enhances the yield but also aligns with sustainable practices in pharmaceutical manufacturing . The following table summarizes key findings from this research:
Enzyme | Function | Yield (%) |
---|---|---|
L-amino acid deaminase | Converts L-phenylalanine to L-Phg | 7.21 |
Leucine dehydrogenase | Bypasses glutamate for amine donor | 99.9 |
Potential Industrial Applications
The production of enantiopure amino acids like this compound is crucial in agrochemical and pharmaceutical industries due to their specific biological activities. The efficient synthesis methods developed could lead to increased availability and reduced costs for these compounds, making them more accessible for research and therapeutic use .
Case Studies
4.1 Case Study: Antidiabetic Activity
A study conducted on various derivatives of this compound revealed that certain compounds exhibited potent inhibition of alpha-glucosidase and dipeptidyl peptidase-IV (DPP-IV), both targets in diabetes management. The findings indicated that these derivatives could serve as lead compounds for developing new antidiabetic therapies with improved efficacy and safety profiles .
4.2 Case Study: Neuropharmacology
In a neuropharmacological study, the application of this compound as an ASCT1/ASCT2 inhibitor highlighted its role in enhancing synaptic plasticity through modulation of d-serine levels. This suggests potential applications in treating cognitive disorders where NMDA receptor function is compromised .
Q & A
Basic Research Questions
Q. What are the primary enzymatic pathways for L-phenylglycine synthesis, and how do they differ in efficiency and stereoselectivity?
this compound is synthesized via two main enzymatic pathways: (1) asymmetric reduction of phenylglyoxylic acid using formate dehydrogenase (FDH) variants , and (2) D-mandelate dehydrogenase (DMDH) coupled with L-leucine dehydrogenase (LeuDH) for cofactor recycling . The FDH pathway achieves >99% enantiomeric excess (e.e.) but requires NADH regeneration, while the DMDH-LeuDH system operates with lower coenzyme concentrations (0.1 mM NAD⁺) and achieves >98% yield and e.e. under optimized conditions . Key challenges include balancing cofactor dependency and substrate inhibition in high-concentration reactions .
Q. How can researchers validate the purity and stereochemical configuration of synthesized this compound?
Techniques include:
- Chiral HPLC : To determine enantiomeric excess (e.g., >99% e.e. achieved via DMDH-LeuDH cascades) .
- ¹³C NMR : For structural confirmation, particularly for distinguishing diastereomeric complexes in enantioselective processes .
- X-ray crystallography : Used to resolve ternary complexes (e.g., macrocycle-amino acid-crown ether systems) and confirm binding geometries . Physicochemical properties (e.g., logP = 1.57, density = 1.178 g/cm³) should align with reference data .
Q. What are the common challenges in scaling up this compound biosynthesis, and how can they be mitigated?
Key issues include:
- Cofactor dependency : Solved by engineering self-sufficient systems (e.g., protein scaffolds linking dehydrogenases and cofactor-regenerating enzymes) .
- Substrate/product inhibition : Addressed via fed-batch strategies or enzyme evolution for higher tolerance (e.g., FDH mutants with improved catalytic efficiency) .
- Low yields in whole-cell systems : Optimized by tuning promoter strength and transporter expression in E. coli .
Advanced Research Questions
Q. How can directed evolution improve the catalytic efficiency of formate dehydrogenase (FDH) for this compound synthesis?
Directed evolution of FDH involves:
- Saturation mutagenesis : Targeting residues near the active site (e.g., positions 245–248) to enhance NADH binding affinity .
- High-throughput screening : Using colorimetric assays (e.g., NADH-dependent reduction of tetrazolium salts) to identify variants with 2–3× higher activity .
- Structural-guided engineering : Incorporating computational docking to predict mutations that stabilize transition states during phenylglyoxylic acid reduction .
Q. What methodologies enable enantioselective separation of D,this compound in non-aqueous systems?
- Liquid membranes with chiral selectors : Chloroform membranes containing cinchona alkaloid derivatives (30 mM) achieve >90% enantioselectivity by preferential transport of D-enantiomers .
- Macrocyclic receptors : TLC plates impregnated with this compound and 18-crown-6 ether resolve racemic mixtures via diastereomeric complex formation, validated by HPLC .
- Crystallization-driven resolution : Co-crystallization with enantiopure macrocycles (e.g., (S)-2) selectively binds D-phenylglycine, confirmed by X-ray diffraction .
Q. How can multi-enzyme cascades optimize this compound production from bio-based precursors?
A four-step cascade converting L-phenylalanine to this compound involves:
- Phenylalanine ammonia-lyase (PAL) : Deaminates L-phenylalanine to cinnamic acid.
- Hydroxylase : Converts cinnamic acid to mandelic acid.
- D-mandelate dehydrogenase : Oxidizes mandelic acid to phenylglyoxylic acid.
- Amino acid dehydrogenase : Reduces phenylglyoxylic acid to this compound with NADH regeneration . This system achieves 99.9% conversion by coupling cofactor recycling and minimizing intermediate toxicity .
Q. What strategies enhance the thermostability and solvent tolerance of this compound-synthesizing enzymes?
- Consensus mutagenesis : Replace unstable residues with consensus amino acids from thermophilic homologs (e.g., Bacillus clausii LeuDH variants) .
- Immobilization : Entrap enzymes in silica-based matrices or covalent organic frameworks (COFs) to retain >80% activity after 10 reaction cycles .
- Solvent engineering : Use ionic liquids (e.g., [BMIM][PF₆]) to stabilize enzymes in biphasic systems, improving substrate solubility .
Q. Methodological Best Practices
Q. How should researchers design experiments to ensure reproducibility in this compound synthesis?
- Documentation : Record enzyme concentrations (e.g., kU/L), NAD⁺ levels (0.1–0.5 mM), and substrate loading (200–500 mM) .
- Control experiments : Include wild-type enzyme comparisons and negative controls (e.g., heat-inactivated enzymes) to confirm activity .
- Data sharing : Deposit raw NMR/HPLC chromatograms in repositories like Zenodo, adhering to FAIR principles .
Q. What computational tools are critical for predicting enzyme-substrate interactions in this compound pathways?
- Molecular docking (AutoDock Vina) : Predict binding modes of phenylglyoxylic acid in FDH active sites .
- MD simulations (GROMACS) : Simulate conformational changes in DMDH during catalysis to identify flexibility hotspots .
- QM/MM calculations : Model transition states for asymmetric reduction steps to guide mutagenesis .
Comparison with Similar Compounds
L-Phenylglycine belongs to a class of aromatic non-proteinogenic amino acids. Below is a comparative analysis with structurally or functionally related compounds:
Table 1: Comparative Analysis of this compound and Analogous Compounds
Compound | Synthesis Method | Key Applications | Enantioselectivity | Advantages/Limitations | References |
---|---|---|---|---|---|
This compound | Microbial bioconversion from L-Phenylalanine or glucose via engineered E. coli pathways | Antibiotics (penicillin), taxol, chiral synthons | >99% ee | High enantiopurity; scalable biocatalysis | [1, 10, 22] |
D-Phenylglycine | Enzymatic resolution of racemic mandelic acid using mandelate racemase (MR) and D-mandelate dehydrogenase (D-MDH) | Semisynthetic antibiotics (e.g., cefalexin) | 97–99% ee | Requires racemization steps; lower yield than L-form | [5, 13, 25] |
4-(Trifluoromethyl)-L-Phenylglycine | Chemical modification of this compound via trifluoromethylation | Enzyme/receptor modulation studies | High chiral purity | Used in research; limited industrial adoption | [21] |
L-Mandelic Acid | Biocatalytic cascade from styrene or racemic mandelic acid via MR and L-amino acid dehydrogenase (L-AADH) | Precursor for this compound; cosmetics | >99% ee after conversion | Requires multi-enzyme systems; scalable | [8, 25] |
Phenylpropionylglycine | Hydrolysis of nitriles using Rhodococcus sp. nitrile hydratase/amidase | Metabolic studies; rare in pharmaceuticals | 48–52% yield (mixed D/L) | Low selectivity; niche applications | [13, 26] |
Key Differences:
Stereoselectivity : this compound is prioritized in drug synthesis due to its compatibility with human biochemistry, whereas D-Phenylglycine is used in specific antibiotics but requires additional resolution steps .
Synthetic Efficiency : Microbial pathways for this compound achieve near-quantitative yields (e.g., 99.9% conversion from L-Phenylalanine) , outperforming chemical methods for analogs like phenylpropionylglycine .
Applications : While L-Mandelic Acid is a precursor, this compound is directly incorporated into APIs, giving it broader industrial relevance .
Key Research Findings
Enhanced Biocatalytic Pathways
- Cofactor Self-Sufficiency : Engineering E. coli with a cofactor regeneration system (GDH-LeuDH scaffold) increased this compound production 5-fold (0.23 mM to 1.15 mM) by recycling NADH and NH₄⁺ .
- Domino Reaction Systems: A three-enzyme cascade (mandelate racemase, D-MDH, and L-AADH) converted 200 mM racemic mandelic acid to this compound with 96.4% yield and >99% ee .
- High-Substrate Loading: Bacillus clausii amino acid dehydrogenase (BcAADH) achieved 400 mM benzoylformic acid conversion to this compound (>99.9% ee) at 60 g·g⁻¹ substrate-to-biocatalyst ratio .
Industrial-Scale Production
- A four-step enzymatic process using glucose or L-Phenylalanine as substrates achieved 99.9% conversion efficiency, demonstrating feasibility for ton-scale manufacturing .
Properties
IUPAC Name |
(2S)-2-amino-2-phenylacetic acid | |
---|---|---|
Source | PubChem | |
URL | https://pubchem.ncbi.nlm.nih.gov | |
Description | Data deposited in or computed by PubChem | |
InChI |
InChI=1S/C8H9NO2/c9-7(8(10)11)6-4-2-1-3-5-6/h1-5,7H,9H2,(H,10,11)/t7-/m0/s1 | |
Source | PubChem | |
URL | https://pubchem.ncbi.nlm.nih.gov | |
Description | Data deposited in or computed by PubChem | |
InChI Key |
ZGUNAGUHMKGQNY-ZETCQYMHSA-N | |
Source | PubChem | |
URL | https://pubchem.ncbi.nlm.nih.gov | |
Description | Data deposited in or computed by PubChem | |
Canonical SMILES |
C1=CC=C(C=C1)C(C(=O)O)N | |
Source | PubChem | |
URL | https://pubchem.ncbi.nlm.nih.gov | |
Description | Data deposited in or computed by PubChem | |
Isomeric SMILES |
C1=CC=C(C=C1)[C@@H](C(=O)O)N | |
Source | PubChem | |
URL | https://pubchem.ncbi.nlm.nih.gov | |
Description | Data deposited in or computed by PubChem | |
Molecular Formula |
C8H9NO2 | |
Source | PubChem | |
URL | https://pubchem.ncbi.nlm.nih.gov | |
Description | Data deposited in or computed by PubChem | |
DSSTOX Substance ID |
DTXSID801022916 | |
Record name | L-Phenylglycine | |
Source | EPA DSSTox | |
URL | https://comptox.epa.gov/dashboard/DTXSID801022916 | |
Description | DSSTox provides a high quality public chemistry resource for supporting improved predictive toxicology. | |
Molecular Weight |
151.16 g/mol | |
Source | PubChem | |
URL | https://pubchem.ncbi.nlm.nih.gov | |
Description | Data deposited in or computed by PubChem | |
CAS No. |
2935-35-5, 69-91-0 | |
Record name | L-Phenylglycine | |
Source | CAS Common Chemistry | |
URL | https://commonchemistry.cas.org/detail?cas_rn=2935-35-5 | |
Description | CAS Common Chemistry is an open community resource for accessing chemical information. Nearly 500,000 chemical substances from CAS REGISTRY cover areas of community interest, including common and frequently regulated chemicals, and those relevant to high school and undergraduate chemistry classes. This chemical information, curated by our expert scientists, is provided in alignment with our mission as a division of the American Chemical Society. | |
Explanation | The data from CAS Common Chemistry is provided under a CC-BY-NC 4.0 license, unless otherwise stated. | |
Record name | Phenylglycine, L- | |
Source | ChemIDplus | |
URL | https://pubchem.ncbi.nlm.nih.gov/substance/?source=chemidplus&sourceid=0002935355 | |
Description | ChemIDplus is a free, web search system that provides access to the structure and nomenclature authority files used for the identification of chemical substances cited in National Library of Medicine (NLM) databases, including the TOXNET system. | |
Record name | Benzeneacetic acid, .alpha.-amino-, (.alpha.S)- | |
Source | EPA Chemicals under the TSCA | |
URL | https://www.epa.gov/chemicals-under-tsca | |
Description | EPA Chemicals under the Toxic Substances Control Act (TSCA) collection contains information on chemicals and their regulations under TSCA, including non-confidential content from the TSCA Chemical Substance Inventory and Chemical Data Reporting. | |
Record name | L-Phenylglycine | |
Source | EPA DSSTox | |
URL | https://comptox.epa.gov/dashboard/DTXSID801022916 | |
Description | DSSTox provides a high quality public chemistry resource for supporting improved predictive toxicology. | |
Record name | L-(+)-α-phenylglycine | |
Source | European Chemicals Agency (ECHA) | |
URL | https://echa.europa.eu/substance-information/-/substanceinfo/100.019.009 | |
Description | The European Chemicals Agency (ECHA) is an agency of the European Union which is the driving force among regulatory authorities in implementing the EU's groundbreaking chemicals legislation for the benefit of human health and the environment as well as for innovation and competitiveness. | |
Explanation | Use of the information, documents and data from the ECHA website is subject to the terms and conditions of this Legal Notice, and subject to other binding limitations provided for under applicable law, the information, documents and data made available on the ECHA website may be reproduced, distributed and/or used, totally or in part, for non-commercial purposes provided that ECHA is acknowledged as the source: "Source: European Chemicals Agency, http://echa.europa.eu/". Such acknowledgement must be included in each copy of the material. ECHA permits and encourages organisations and individuals to create links to the ECHA website under the following cumulative conditions: Links can only be made to webpages that provide a link to the Legal Notice page. | |
Record name | α-phenylglycine | |
Source | European Chemicals Agency (ECHA) | |
URL | https://echa.europa.eu/substance-information/-/substanceinfo/100.000.654 | |
Description | The European Chemicals Agency (ECHA) is an agency of the European Union which is the driving force among regulatory authorities in implementing the EU's groundbreaking chemicals legislation for the benefit of human health and the environment as well as for innovation and competitiveness. | |
Explanation | Use of the information, documents and data from the ECHA website is subject to the terms and conditions of this Legal Notice, and subject to other binding limitations provided for under applicable law, the information, documents and data made available on the ECHA website may be reproduced, distributed and/or used, totally or in part, for non-commercial purposes provided that ECHA is acknowledged as the source: "Source: European Chemicals Agency, http://echa.europa.eu/". Such acknowledgement must be included in each copy of the material. ECHA permits and encourages organisations and individuals to create links to the ECHA website under the following cumulative conditions: Links can only be made to webpages that provide a link to the Legal Notice page. | |
Record name | PHENYLGLYCINE, L- | |
Source | FDA Global Substance Registration System (GSRS) | |
URL | https://gsrs.ncats.nih.gov/ginas/app/beta/substances/3I753R0XI6 | |
Description | The FDA Global Substance Registration System (GSRS) enables the efficient and accurate exchange of information on what substances are in regulated products. Instead of relying on names, which vary across regulatory domains, countries, and regions, the GSRS knowledge base makes it possible for substances to be defined by standardized, scientific descriptions. | |
Explanation | Unless otherwise noted, the contents of the FDA website (www.fda.gov), both text and graphics, are not copyrighted. They are in the public domain and may be republished, reprinted and otherwise used freely by anyone without the need to obtain permission from FDA. Credit to the U.S. Food and Drug Administration as the source is appreciated but not required. | |
Retrosynthesis Analysis
AI-Powered Synthesis Planning: Our tool employs the Template_relevance Pistachio, Template_relevance Bkms_metabolic, Template_relevance Pistachio_ringbreaker, Template_relevance Reaxys, Template_relevance Reaxys_biocatalysis model, leveraging a vast database of chemical reactions to predict feasible synthetic routes.
One-Step Synthesis Focus: Specifically designed for one-step synthesis, it provides concise and direct routes for your target compounds, streamlining the synthesis process.
Accurate Predictions: Utilizing the extensive PISTACHIO, BKMS_METABOLIC, PISTACHIO_RINGBREAKER, REAXYS, REAXYS_BIOCATALYSIS database, our tool offers high-accuracy predictions, reflecting the latest in chemical research and data.
Strategy Settings
Precursor scoring | Relevance Heuristic |
---|---|
Min. plausibility | 0.01 |
Model | Template_relevance |
Template Set | Pistachio/Bkms_metabolic/Pistachio_ringbreaker/Reaxys/Reaxys_biocatalysis |
Top-N result to add to graph | 6 |
Feasible Synthetic Routes
Disclaimer and Information on In-Vitro Research Products
Please be aware that all articles and product information presented on BenchChem are intended solely for informational purposes. The products available for purchase on BenchChem are specifically designed for in-vitro studies, which are conducted outside of living organisms. In-vitro studies, derived from the Latin term "in glass," involve experiments performed in controlled laboratory settings using cells or tissues. It is important to note that these products are not categorized as medicines or drugs, and they have not received approval from the FDA for the prevention, treatment, or cure of any medical condition, ailment, or disease. We must emphasize that any form of bodily introduction of these products into humans or animals is strictly prohibited by law. It is essential to adhere to these guidelines to ensure compliance with legal and ethical standards in research and experimentation.