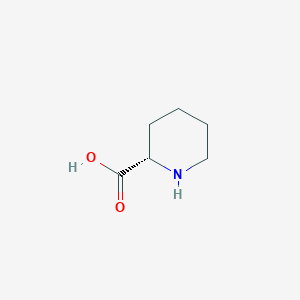
l-Pipecolic acid
Overview
Description
L-Pipecolic acid (L-Pip), also known as L-homoproline or piperidine-2-carboxylic acid, is a cyclic non-proteinogenic amino acid and a key intermediate in lysine catabolism . It is synthesized via the lysine cyclodeaminase (LCD)-catalyzed β-deamination of L-lysine, utilizing β-nicotinamide adenine dinucleotide (NAD⁺) as a cofactor . L-Pip serves as a precursor for piperidine alkaloids, immunosuppressants (e.g., rapamycin), and other bioactive molecules . In humans, its oxidation occurs in peroxisomes via this compound oxidase (PIPOX), producing L-α-aminoadipate δ-semialdehyde (AAA) and hydrogen peroxide (H₂O₂) . Elevated L-Pip levels are associated with peroxisome biogenesis disorders (PBDs) such as Zellweger syndrome, where PIPOX activity is deficient .
Mechanism of Action
l-Pipecolic acid exerts its effects through its involvement in metabolic pathways. It is converted from lysine via enzymatic reactions involving lysine aminotransferase and pyrroline-5-carboxylate reductase. The compound acts as a precursor to several bioactive molecules, influencing various biological processes. For instance, in plants, it plays a role in systemic acquired resistance by regulating free radicals and signaling molecules .
Comparison with Similar Compounds
Comparison with Structurally and Functionally Similar Compounds
L-Pip shares structural and metabolic similarities with several compounds, including L-proline , D-pipecolic acid , and sarcosine . Below is a detailed analysis:
Structural Comparison
Metabolic Pathways and Enzymatic Specificity
- This compound :
- L-Proline :
- D-Pipecolic Acid: Not a substrate for human PIPOX; minimal metabolic relevance in mammals .
- Sarcosine: Oxidized by monomeric sarcosine oxidases (MSOX), which share homology with PIPOX but act on distinct substrates .
Functional and Clinical Differences
- L-Proline metabolism defects cause hyperprolinemia, associated with seizures and cognitive impairment .
- Immune Modulation: L-Pip enhances systemic resistance to bacterial infections via nitric oxide (NO) and reactive oxygen species (ROS) induction in plants . In rheumatoid arthritis (RA), higher L-Pip levels correlate with reduced inflammation, suggesting a role in disease remission .
- Neurological Effects: L-Pip induces hypnotic effects in chicks via GABA receptors, unlike L-proline, which acts on NMDA receptors .
Key Research Findings
Enzymatic Homology: Human PIPOX shares 30–40% sequence identity with bacterial monomeric sarcosine oxidases (MSOX) but <20% with D-amino acid oxidases (DAO) . PIPOX and MSOX both utilize FAD as a cofactor and produce H₂O₂ .
Peroxisomal vs. Mitochondrial Localization :
- In primates, L-Pip oxidation is peroxisomal, while in rabbits, it occurs in mitochondria, highlighting species-specific metabolic adaptations .
Biotechnological Production: Recombinant E. coli expressing LCD achieves 61 g/L L-Pip from glucose, with a 29.6% conversion efficiency .
Biological Activity
L-Pipecolic acid (L-PA) is a cyclic imino acid derived from lysine, which has garnered attention for its diverse biological activities and potential therapeutic applications. This article explores the biochemical properties, metabolic pathways, and various biological effects of this compound, supported by case studies and research findings.
1. Chemical Structure and Properties
This compound is characterized by its six-membered ring structure, which is a derivative of the amino acid lysine. It exists in two enantiomeric forms: D-pipecolic acid and this compound, with the latter being more biologically active in several contexts.
2. Metabolism and Biochemical Role
This compound is primarily produced through the catabolism of lysine by intestinal bacteria and is also synthesized in mammals. It plays a significant role in various metabolic processes:
- Peroxisomal Function : L-PA is oxidized in peroxisomes to form alpha-aminoadipic acid (AAA), a process that is deficient in certain genetic disorders such as Zellweger syndrome. In healthy liver tissues, L-PA oxidation rates are significantly higher compared to those in affected individuals, highlighting its role in metabolic health .
- Compatible Solute : In Corynebacterium glutamicum, L-PA functions as a compatible solute that aids in osmotic stress tolerance. This organism accumulates L-PA under hyper-osmotic conditions, which helps maintain cellular integrity during osmotic fluctuations .
3.1 Neuropharmacological Effects
Research indicates that this compound exhibits hypnotic effects when administered centrally in animal models. Studies show that it does not activate N-methyl-D-aspartate receptors but may influence sleep patterns through other mechanisms .
3.2 Antimicrobial Properties
This compound has demonstrated antibacterial activity against various pathogens. For instance, it is an essential component of xylapeptide A, which shows strong antibacterial effects against Bacillus subtilis and Staphylococcus aureus with minimal inhibitory concentrations (MIC) as low as 6.25 μg/mL . This suggests potential applications in developing antimicrobial agents.
3.3 Plant Immunity
In plants, this compound is implicated in systemic acquired resistance (SAR) against pathogens. It regulates immune responses and enhances resistance to bacterial infections, making it a critical compound for plant defense mechanisms .
4. Research Findings and Case Studies
The following table summarizes key studies on the biological activity of this compound:
5. Conclusion
This compound is a multifaceted compound with significant biological activities across various domains, including human health, plant biology, and microbial interactions. Its role in metabolic pathways highlights its importance in both health and disease contexts, particularly concerning genetic disorders affecting peroxisomal function. Ongoing research into its pharmacological potentials may pave the way for novel therapeutic applications.
Scientific Research Applications
Biochemical Applications
L-Pipecolic acid plays a significant role in metabolic pathways and has been implicated in various physiological processes.
Metabolism and Enzymatic Activity
This compound is oxidized in the liver through the action of L-pipecolate oxidase, an enzyme located in peroxisomes and mitochondria. This oxidation process produces hydrogen peroxide and L-alpha-aminoadipate delta-semialdehyde as immediate products. Studies have shown that this enzymatic activity varies with age and tissue type, highlighting its importance in metabolic regulation .
Plant Immunity
Recent research indicates that this compound is crucial for systemic acquired resistance in plants. It acts as a signaling molecule that enhances plant immunity against bacterial pathogens, thereby improving plant health and resilience . This application could be pivotal in agricultural biotechnology for developing disease-resistant crops.
Medical Applications
This compound has been studied for its therapeutic potential in various medical conditions.
Neurological Disorders
Elevated levels of this compound have been associated with neurological disorders such as Zellweger syndrome and other peroxisomal disorders. The accumulation of this metabolite serves as a biomarker for diagnosing these conditions . Understanding its role in these disorders could lead to better diagnostic tools and potential therapies.
Gut Health
Recent studies have demonstrated that this compound can alleviate symptoms of functional constipation by modulating gut microbiota composition and enhancing intestinal transit rates. It was found to increase serotonin levels in the serum, which is crucial for gut motility . This suggests potential applications in treating gastrointestinal disorders.
Microbiological Applications
This compound's interaction with microbiota has implications for health and disease.
Microbial Metabolism
Research indicates that certain gut bacteria metabolize this compound, influencing overall gut health. For instance, alterations in the abundance of specific bacteria were correlated with serum levels of this compound in children with functional constipation . This highlights the compound's role as a metabolic substrate that can affect microbial community dynamics.
Osmotic Stress Response
In microbial systems, particularly in Corynebacterium glutamicum, this compound has been shown to function as an osmoprotectant under hyperosmotic stress conditions. This property enhances microbial growth and stability, suggesting its utility in biotechnology applications where osmotic stress is a concern .
Case Studies
Chemical Reactions Analysis
Lysine Cyclodeaminase (LCD) Pathway
- Reaction : L-Lysine → L-PA via a single enzymatic step (deamination and cyclization) .
- Key Enzymes : LCD from Streptomyces hygroscopicus (RapL) .
Saccharopine Pathway
- Steps :
Biochemical Role : L-PA regulates systemic acquired resistance (SAR) in plants by amplifying defense signals against pathogens .
Cell-Free Systems
- Dual-Enzyme System : Lysine-6-dehydrogenase (Ly6DH) and pyrroline-5-carboxylate reductase (P5CR) convert L-lysine to L-PA with >99% conversion in batch reactions. Co-immobilized enzymes in flow reactors achieve 2.5 g L⁻¹ h⁻¹ space-time yield .
- Transaminase Route : ε-Amino group transamination of L-lysine followed by cyclization and reduction yields L-PA (60% conversion) .
Table 1 : Key Biocatalytic Systems for L-PA Synthesis
Enzymes | Cofactor | Conversion | Yield | Ref |
---|---|---|---|---|
Ly6DH + P5CR | NADH | >99% | 50 mM | |
Transaminase + P5CR | NADPH | 60% | 50 mM | |
LCD (RapL) | – | 95% | 61 g/L |
Industrial Production via Metabolic Engineering
- Microbial Fermentation : Engineered E. coli strains with lysine riboswitches and multiple pipA (LCD) gene copies produce 61 g/L L-PA (29.6% glucose conversion) .
- Key Modifications : Dynamic regulation of lysine flux and enzyme expression using CIChE (chemically induced chromosomal evolution) .
From Picolinic Acid
Reactivity in Coordination Chemistry
L-PA forms stable complexes with transition metals:
- Copper Complex : Cu(L-PA)₂(H₂O)₂ exhibits square-planar geometry, with carboxylate and amine groups acting as ligands .
Degradation and Metabolic Fate
- Oxidation : In human liver peroxisomes, L-PA is oxidized to L-α-aminoadipic acid via L-pipecolate oxidase, generating H₂O₂ .
- Bacterial Catabolism : Gut microbiota convert dietary lysine to D- and L-PA, influencing plasma levels .
Key Data Tables
Table 2 : Physicochemical Properties of this compound
Property | Value | Ref |
---|---|---|
Molecular Formula | C₆H₁₁NO₂ | |
Molecular Weight | 129.16 g/mol | |
pKa (Strongest Acidic) | 2.06 | |
pKa (Strongest Basic) | 10.39 | |
Melting Point | 268–270°C (decomposes) |
Table 3 : Applications of L-PA in Synthesis
Q & A
Basic Research Questions
Q. What are the primary metabolic pathways involving L-pipecolic acid in humans, and how can researchers analyze these pathways experimentally?
this compound is a product of lysine catabolism, primarily via the saccharopine pathway. In humans, peroxisomal oxidation of this compound to α-aminoadipic acid is critical, as deficiencies in this process are linked to Zellweger syndrome . To analyze these pathways, researchers can:
- Use isotopic labeling (e.g., L-[³H]pipecolic acid) in tissue homogenates to track metabolic flux .
- Perform enzyme activity assays for δ1-piperideine-2-carboxylate reductase and peroxisomal this compound oxidase .
- Compare metabolite levels in plasma or cerebrospinal fluid from patients with peroxisomal disorders versus controls using GC-MS or LC-MS/MS .
Q. What standard methods are used to detect and quantify this compound in biological samples?
- Chromatography : GC-MS and LC-MS/MS are gold standards, with derivatization (e.g., trimethylsilylation) enhancing sensitivity .
- Enzymatic assays : Coupled reactions with NADH-dependent enzymes to measure oxidation products .
- Isotopic tracing : Radiolabeled this compound (³H or ¹⁴C) in cell cultures or animal models to study turnover rates .
Advanced Research Questions
Q. How can researchers reconcile contradictory findings regarding this compound’s dual roles in oxidative stress and inflammation?
this compound exhibits pro-inflammatory effects (e.g., increasing reactive oxygen species in plant defense) but anti-inflammatory potential in rheumatoid arthritis (RA) remission . To address contradictions:
- Context-specific models : Use tissue-specific assays (e.g., cerebral cortex vs. synovial fluid) to evaluate oxidative stress markers (e.g., nitric oxide, lipid peroxidation) .
- Longitudinal clinical studies : Compare this compound levels in RA patients pre/post immunosuppressive therapy to assess its role as a remission biomarker .
- Mechanistic studies : Investigate signaling pathways (e.g., NF-κB) in macrophages exposed to this compound under varying inflammatory conditions .
Q. What genetic engineering strategies improve microbial production of this compound from glucose?
- Dynamic regulation : Use lysine riboswitches to control substrate (L-lysine) availability, optimizing flux toward this compound .
- Gene amplification : Employ chemically inducible chromosomal evolution (CIChE) to integrate multiple copies of pipA (lysine cyclodeaminase) into E. coli genomes .
- Strain optimization : Combine high-lysine-producing strains (e.g., Corynebacterium glutamicum) with heterologous pipA expression for higher titers (e.g., 61 g/L in fed-batch fermentation) .
Q. How can researchers design experiments to study this compound’s role in peroxisomal disorders like Zellweger syndrome?
- Peroxisome isolation : Fractionate liver mitochondrial/peroxisomal extracts via Percoll gradients, correlating this compound oxidation with catalase activity (a peroxisomal marker) .
- Knockout models : Use CRISPR-Cas9 to disrupt peroxisomal oxidase genes in human cell lines and measure metabolite accumulation .
- Clinical metabolomics : Profile this compound levels in patient serum using NMR or mass spectrometry to establish diagnostic thresholds .
Q. Methodological Considerations
- Contradiction resolution : When conflicting data arise (e.g., neurotoxicity vs. anti-inflammatory effects), prioritize in vivo models that mimic human disease states over isolated in vitro systems .
- Ethical compliance : For clinical studies, adhere to protocols for handling biohazardous waste from this compound experiments, including professional disposal of contaminated materials .
Preparation Methods
Chemical Synthesis and Resolution Methods
Diastereomeric Salt Resolution Using Tartaric Acids
The resolution of racemic DL-pipecolic acid via diastereomeric salt formation remains a cornerstone of industrial production. In the method disclosed in US7683175B2, Dthis compound is treated with L-tartaric acid in ethanol-water (95:5) at 80°C to preferentially crystallize the this compound-L-tartrate complex . Subsequent recrystallization with D-tartaric acid removes residual D-enantiomer impurities, yielding L-PA with >99.5% optical purity. Critical parameters include solvent polarity (ethanol-water mixtures) and stoichiometric excess of tartaric acid (1.2 equiv) . However, this method generates stoichiometric tartaric acid waste, necessitating costly recycling processes.
A modified approach in WO2009089842A1 employs dibenzoyl-L-tartaric acid for resolving intermediates like pipecolic-2-acid-2',6'-xylidide . Here, the L-enantiomer forms an insoluble salt in isopropanol, achieving 90–92% chiral purity after initial crystallization. Further purification via toluene recrystallization enhances enantiomeric excess to >99%, albeit with a 40% overall yield . These resolution methods are limited by low atom economy and high solvent consumption, driving interest in asymmetric synthesis.
Multi-Step Organic Synthesis from Chiral Precursors
Fleet’s 1986 synthesis of 4,5-dihydroxy-L-pipecolic acid from D-glucoronolactone exemplifies chiral pool strategies . The 12-step route begins with triflation of D-glucoronolactone, followed by azide displacement and catalytic hydrogenation to install the piperidine ring (Scheme 11) . Key stereochemical outcomes arise from substrate-controlled reductions, preserving the D-glucose-derived configuration. Despite meticulous stereocontrol, the synthesis suffers from modest yields (15–20% overall) due to multiple protection-deprotection steps .
Alternative routes leverage cyclic imine intermediates. For instance, TrHN-protected methyl esters undergo conjugate addition with aldehydes (RCHO) in acetonitrile, followed by chemoselective reduction to yield 4-oxo-L-pipecolic acid derivatives (Scheme 2) . Deprotection under acidic conditions (HCl) furnishes 6-substituted 4-hydroxy-L-pipecolic acids in 29–53% yield over four steps . While these methods enable structural diversification, they remain impractical for bulk production due to lengthy sequences and hazardous reagents (e.g., triflic anhydride).
Biocatalytic and Enzymatic Production Strategies
Cell-Free Enzyme Systems with Cofactor Recycling
Cell-free biocatalysis offers a green alternative to chemical synthesis. A bienzymatic system combining lysine-6-dehydrogenase (L6DH) and pyrroline-5-carboxylate reductase (P5CR) converts L-lysine directly to L-PA with >99% conversion in batch mode . The redox-neutral cascade operates at pH 10, utilizing catalytic NADH (0.1 equiv) regenerated by formate dehydrogenase (FDH) . Key advantages include:
-
Elimination of side products : L6DH’s strict regioselectivity for ε-deamination prevents α-deamination pathways .
-
Solvent tolerance : Enzymes remain active in 20% (v/v) acetone, facilitating product precipitation .
At 50 mM substrate loading, this system achieves a space-time yield of 2.5 g L h in continuous flow reactors using co-immobilized enzymes on EziG carriers . Process intensification reduces cofactor costs by 90% compared to free-enzyme batches .
Immobilized Enzyme Reactors in Continuous Flow
Immobilization of L6DH and P5CR on porous silica (EziG^® OPAL) enhances operational stability, enabling 30-day continuous operation without activity loss . The packed-bed reactor configuration (30 min residence time) achieves >99% conversion at 100 mM L-lysine input, outperforming batch systems in productivity (Table 1) .
Table 1: Performance of Immobilized vs. Free Enzyme Systems
Parameter | Free Enzymes (Batch) | Immobilized (Flow) |
---|---|---|
Conversion (%) | >99 | >99 |
Space-Time Yield | 1.2 g L h | 2.5 g L h |
Cofactor Loading | 0.1 equiv | 0.01 equiv |
Operational Stability | 3 cycles | 30 days |
Metabolic Engineering and Microbial Fermentation
Pathway Optimization in Saccharomyces cerevisiae
Metabolic engineering of S. cerevisiae BY4743 enables de novo L-PA synthesis from glucose . The optimized pathway includes:
-
Lysine ε-deaminase (lysine → Δ-piperidine-6-carboxylate)
-
Piperideine-6-carboxylate reductase (Δ-P6C → L-PA)
Global transcription machinery engineering (gTME) and Kozak sequence modifications enhance enzyme expression, boosting titers from 0.60 g/L to 5.47 g/L in shake flasks .
Fed-Batch Fermentation for High-Yield Production
Scaling to a 5 L bioreactor with glucose feeding achieves 74.54 g/L L-PA, the highest reported titer to date . Key parameters include:
-
Dissolved oxygen : Maintained at 30% saturation to prevent acetate accumulation.
-
pH control : 6.0 via NHOH addition to stabilize reductase activity .
Comparative Analysis of Preparation Methods
Table 2: Method Comparison for L-PA Production
Method | Yield (%) | ee (%) | Scalability | Green Metrics |
---|---|---|---|---|
Tartaric Acid Resolution | 40–60 | >99.5 | Moderate | Low (solvent waste) |
Biocatalytic Flow | >99 | >99 | High | High (cofactor recycling) |
Microbial Fermentation | 74.54 g/L | >99 | Industrial | Moderate (feedstock use) |
Properties
IUPAC Name |
piperidine-2-carboxylic acid | |
---|---|---|
Source | PubChem | |
URL | https://pubchem.ncbi.nlm.nih.gov | |
Description | Data deposited in or computed by PubChem | |
InChI |
InChI=1S/C6H11NO2/c8-6(9)5-3-1-2-4-7-5/h5,7H,1-4H2,(H,8,9) | |
Source | PubChem | |
URL | https://pubchem.ncbi.nlm.nih.gov | |
Description | Data deposited in or computed by PubChem | |
InChI Key |
HXEACLLIILLPRG-UHFFFAOYSA-N | |
Source | PubChem | |
URL | https://pubchem.ncbi.nlm.nih.gov | |
Description | Data deposited in or computed by PubChem | |
Canonical SMILES |
C1CCNC(C1)C(=O)O | |
Source | PubChem | |
URL | https://pubchem.ncbi.nlm.nih.gov | |
Description | Data deposited in or computed by PubChem | |
Molecular Formula |
C6H11NO2 | |
Source | PubChem | |
URL | https://pubchem.ncbi.nlm.nih.gov | |
Description | Data deposited in or computed by PubChem | |
Related CAS |
69470-51-5 (mono-potassium salt), 15862-86-9 (hydrochloride salt/solvate) | |
Record name | Pipecolic acid | |
Source | ChemIDplus | |
URL | https://pubchem.ncbi.nlm.nih.gov/substance/?source=chemidplus&sourceid=0000535751 | |
Description | ChemIDplus is a free, web search system that provides access to the structure and nomenclature authority files used for the identification of chemical substances cited in National Library of Medicine (NLM) databases, including the TOXNET system. | |
DSSTOX Substance ID |
DTXSID40862144 | |
Record name | 2-Piperidinecarboxylic acid | |
Source | EPA DSSTox | |
URL | https://comptox.epa.gov/dashboard/DTXSID40862144 | |
Description | DSSTox provides a high quality public chemistry resource for supporting improved predictive toxicology. | |
Molecular Weight |
129.16 g/mol | |
Source | PubChem | |
URL | https://pubchem.ncbi.nlm.nih.gov | |
Description | Data deposited in or computed by PubChem | |
Physical Description |
Solid | |
Record name | Pipecolic acid | |
Source | Human Metabolome Database (HMDB) | |
URL | http://www.hmdb.ca/metabolites/HMDB0000070 | |
Description | The Human Metabolome Database (HMDB) is a freely available electronic database containing detailed information about small molecule metabolites found in the human body. | |
Explanation | HMDB is offered to the public as a freely available resource. Use and re-distribution of the data, in whole or in part, for commercial purposes requires explicit permission of the authors and explicit acknowledgment of the source material (HMDB) and the original publication (see the HMDB citing page). We ask that users who download significant portions of the database cite the HMDB paper in any resulting publications. | |
Solubility |
314 mg/mL | |
Record name | Pipecolic acid | |
Source | Human Metabolome Database (HMDB) | |
URL | http://www.hmdb.ca/metabolites/HMDB0000070 | |
Description | The Human Metabolome Database (HMDB) is a freely available electronic database containing detailed information about small molecule metabolites found in the human body. | |
Explanation | HMDB is offered to the public as a freely available resource. Use and re-distribution of the data, in whole or in part, for commercial purposes requires explicit permission of the authors and explicit acknowledgment of the source material (HMDB) and the original publication (see the HMDB citing page). We ask that users who download significant portions of the database cite the HMDB paper in any resulting publications. | |
CAS No. |
535-75-1, 3105-95-1, 4043-87-2 | |
Record name | Pipecolic acid | |
Source | CAS Common Chemistry | |
URL | https://commonchemistry.cas.org/detail?cas_rn=535-75-1 | |
Description | CAS Common Chemistry is an open community resource for accessing chemical information. Nearly 500,000 chemical substances from CAS REGISTRY cover areas of community interest, including common and frequently regulated chemicals, and those relevant to high school and undergraduate chemistry classes. This chemical information, curated by our expert scientists, is provided in alignment with our mission as a division of the American Chemical Society. | |
Explanation | The data from CAS Common Chemistry is provided under a CC-BY-NC 4.0 license, unless otherwise stated. | |
Record name | Pipecolic acid | |
Source | ChemIDplus | |
URL | https://pubchem.ncbi.nlm.nih.gov/substance/?source=chemidplus&sourceid=0000535751 | |
Description | ChemIDplus is a free, web search system that provides access to the structure and nomenclature authority files used for the identification of chemical substances cited in National Library of Medicine (NLM) databases, including the TOXNET system. | |
Record name | Pipecolic acid | |
Source | DTP/NCI | |
URL | https://dtp.cancer.gov/dtpstandard/servlet/dwindex?searchtype=NSC&outputformat=html&searchlist=93089 | |
Description | The NCI Development Therapeutics Program (DTP) provides services and resources to the academic and private-sector research communities worldwide to facilitate the discovery and development of new cancer therapeutic agents. | |
Explanation | Unless otherwise indicated, all text within NCI products is free of copyright and may be reused without our permission. Credit the National Cancer Institute as the source. | |
Record name | Pipecolic acid | |
Source | DTP/NCI | |
URL | https://dtp.cancer.gov/dtpstandard/servlet/dwindex?searchtype=NSC&outputformat=html&searchlist=17125 | |
Description | The NCI Development Therapeutics Program (DTP) provides services and resources to the academic and private-sector research communities worldwide to facilitate the discovery and development of new cancer therapeutic agents. | |
Explanation | Unless otherwise indicated, all text within NCI products is free of copyright and may be reused without our permission. Credit the National Cancer Institute as the source. | |
Record name | 2-Piperidinecarboxylic acid | |
Source | EPA Chemicals under the TSCA | |
URL | https://www.epa.gov/chemicals-under-tsca | |
Description | EPA Chemicals under the Toxic Substances Control Act (TSCA) collection contains information on chemicals and their regulations under TSCA, including non-confidential content from the TSCA Chemical Substance Inventory and Chemical Data Reporting. | |
Record name | 2-Piperidinecarboxylic acid | |
Source | EPA DSSTox | |
URL | https://comptox.epa.gov/dashboard/DTXSID40862144 | |
Description | DSSTox provides a high quality public chemistry resource for supporting improved predictive toxicology. | |
Record name | DL-piperidine-2-carboxylic acid | |
Source | European Chemicals Agency (ECHA) | |
URL | https://echa.europa.eu/substance-information/-/substanceinfo/100.021.580 | |
Description | The European Chemicals Agency (ECHA) is an agency of the European Union which is the driving force among regulatory authorities in implementing the EU's groundbreaking chemicals legislation for the benefit of human health and the environment as well as for innovation and competitiveness. | |
Explanation | Use of the information, documents and data from the ECHA website is subject to the terms and conditions of this Legal Notice, and subject to other binding limitations provided for under applicable law, the information, documents and data made available on the ECHA website may be reproduced, distributed and/or used, totally or in part, for non-commercial purposes provided that ECHA is acknowledged as the source: "Source: European Chemicals Agency, http://echa.europa.eu/". Such acknowledgement must be included in each copy of the material. ECHA permits and encourages organisations and individuals to create links to the ECHA website under the following cumulative conditions: Links can only be made to webpages that provide a link to the Legal Notice page. | |
Record name | Piperidine-2-carboxylic acid | |
Source | European Chemicals Agency (ECHA) | |
URL | https://echa.europa.eu/substance-information/-/substanceinfo/100.007.835 | |
Description | The European Chemicals Agency (ECHA) is an agency of the European Union which is the driving force among regulatory authorities in implementing the EU's groundbreaking chemicals legislation for the benefit of human health and the environment as well as for innovation and competitiveness. | |
Explanation | Use of the information, documents and data from the ECHA website is subject to the terms and conditions of this Legal Notice, and subject to other binding limitations provided for under applicable law, the information, documents and data made available on the ECHA website may be reproduced, distributed and/or used, totally or in part, for non-commercial purposes provided that ECHA is acknowledged as the source: "Source: European Chemicals Agency, http://echa.europa.eu/". Such acknowledgement must be included in each copy of the material. ECHA permits and encourages organisations and individuals to create links to the ECHA website under the following cumulative conditions: Links can only be made to webpages that provide a link to the Legal Notice page. | |
Record name | PIPECOLIC ACID | |
Source | FDA Global Substance Registration System (GSRS) | |
URL | https://gsrs.ncats.nih.gov/ginas/app/beta/substances/H254GW7PVV | |
Description | The FDA Global Substance Registration System (GSRS) enables the efficient and accurate exchange of information on what substances are in regulated products. Instead of relying on names, which vary across regulatory domains, countries, and regions, the GSRS knowledge base makes it possible for substances to be defined by standardized, scientific descriptions. | |
Explanation | Unless otherwise noted, the contents of the FDA website (www.fda.gov), both text and graphics, are not copyrighted. They are in the public domain and may be republished, reprinted and otherwise used freely by anyone without the need to obtain permission from FDA. Credit to the U.S. Food and Drug Administration as the source is appreciated but not required. | |
Record name | Pipecolic acid | |
Source | Human Metabolome Database (HMDB) | |
URL | http://www.hmdb.ca/metabolites/HMDB0000070 | |
Description | The Human Metabolome Database (HMDB) is a freely available electronic database containing detailed information about small molecule metabolites found in the human body. | |
Explanation | HMDB is offered to the public as a freely available resource. Use and re-distribution of the data, in whole or in part, for commercial purposes requires explicit permission of the authors and explicit acknowledgment of the source material (HMDB) and the original publication (see the HMDB citing page). We ask that users who download significant portions of the database cite the HMDB paper in any resulting publications. | |
Melting Point |
264 °C | |
Record name | Pipecolic acid | |
Source | Human Metabolome Database (HMDB) | |
URL | http://www.hmdb.ca/metabolites/HMDB0000070 | |
Description | The Human Metabolome Database (HMDB) is a freely available electronic database containing detailed information about small molecule metabolites found in the human body. | |
Explanation | HMDB is offered to the public as a freely available resource. Use and re-distribution of the data, in whole or in part, for commercial purposes requires explicit permission of the authors and explicit acknowledgment of the source material (HMDB) and the original publication (see the HMDB citing page). We ask that users who download significant portions of the database cite the HMDB paper in any resulting publications. | |
Retrosynthesis Analysis
AI-Powered Synthesis Planning: Our tool employs the Template_relevance Pistachio, Template_relevance Bkms_metabolic, Template_relevance Pistachio_ringbreaker, Template_relevance Reaxys, Template_relevance Reaxys_biocatalysis model, leveraging a vast database of chemical reactions to predict feasible synthetic routes.
One-Step Synthesis Focus: Specifically designed for one-step synthesis, it provides concise and direct routes for your target compounds, streamlining the synthesis process.
Accurate Predictions: Utilizing the extensive PISTACHIO, BKMS_METABOLIC, PISTACHIO_RINGBREAKER, REAXYS, REAXYS_BIOCATALYSIS database, our tool offers high-accuracy predictions, reflecting the latest in chemical research and data.
Strategy Settings
Precursor scoring | Relevance Heuristic |
---|---|
Min. plausibility | 0.01 |
Model | Template_relevance |
Template Set | Pistachio/Bkms_metabolic/Pistachio_ringbreaker/Reaxys/Reaxys_biocatalysis |
Top-N result to add to graph | 6 |
Feasible Synthetic Routes
Disclaimer and Information on In-Vitro Research Products
Please be aware that all articles and product information presented on BenchChem are intended solely for informational purposes. The products available for purchase on BenchChem are specifically designed for in-vitro studies, which are conducted outside of living organisms. In-vitro studies, derived from the Latin term "in glass," involve experiments performed in controlled laboratory settings using cells or tissues. It is important to note that these products are not categorized as medicines or drugs, and they have not received approval from the FDA for the prevention, treatment, or cure of any medical condition, ailment, or disease. We must emphasize that any form of bodily introduction of these products into humans or animals is strictly prohibited by law. It is essential to adhere to these guidelines to ensure compliance with legal and ethical standards in research and experimentation.