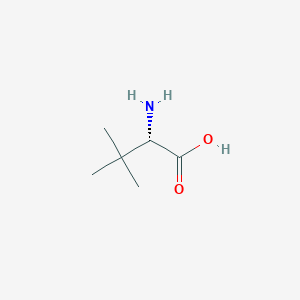
L-tert-Leucine
Overview
Description
L-tert-Leucine is a non-proteinogenic chiral amino acid with significant applications in the pharmaceutical industry. It serves as a chiral intermediate in the synthesis of various drugs, including anticancer and antiviral medications . This compound is particularly valued for its hydrophobic and bulky tert-butyl side chain, which imparts unique properties to the molecules it is incorporated into .
Biochemical Analysis
Biochemical Properties
L-tert-Leucine is extensively used as a crucial building block for chiral auxiliaries, pharmaceutically active ingredients, and ligands . It interacts with enzymes such as leucine dehydrogenase (LeuDH) and formate dehydrogenase (FDH) for regenerating the expensive coenzyme NADH . LeuDH is continually used for synthesizing this compound from α-keto acid .
Molecular Mechanism
At the molecular level, this compound exerts its effects through binding interactions with biomolecules, enzyme inhibition or activation, and changes in gene expression . For instance, LeuDH and FDH are used in the synthesis of this compound, indicating a binding interaction with these enzymes .
Metabolic Pathways
This compound is involved in metabolic pathways that include enzymes or cofactors such as LeuDH and FDH . The compound also affects metabolic flux or metabolite levels .
Preparation Methods
Synthetic Routes and Reaction Conditions: L-tert-Leucine can be synthesized through both chemical and enzymatic methods. The chemical synthesis typically involves the production of racemic Dthis compound, followed by optical resolution to obtain the desired enantiomer . Enzymatic synthesis, on the other hand, employs leucine dehydrogenase to catalyze the asymmetric reduction of trimethylpyruvate .
Industrial Production Methods: In industrial settings, the enzymatic method is preferred due to its high enantioselectivity and environmentally friendly nature. The process involves the use of leucine dehydrogenase and glucose dehydrogenase in a coupled reaction system, which enhances the yield and stability of the enzymes . Additionally, immobilization techniques, such as using zeolite imidazolate frameworks, have been developed to further improve enzyme performance and reusability .
Chemical Reactions Analysis
Types of Reactions: L-tert-Leucine undergoes various chemical reactions, including:
Oxidation: Conversion to corresponding keto acids.
Reduction: Asymmetric reduction of trimethylpyruvate to this compound.
Substitution: Formation of derivatives through substitution reactions
Common Reagents and Conditions:
Oxidation: Typically involves oxidizing agents like potassium permanganate.
Reduction: Utilizes NADH-dependent leucine dehydrogenase.
Substitution: Employs various nucleophiles under mild conditions.
Major Products:
Oxidation: Keto acids.
Reduction: this compound.
Substitution: Various this compound derivatives
Scientific Research Applications
L-tert-Leucine is extensively used in scientific research due to its role as a chiral building block. Its applications include:
Chemistry: Used as a chiral auxiliary in asymmetric synthesis.
Biology: Serves as a precursor for the synthesis of biologically active molecules.
Medicine: Integral in the production of anticancer and antiviral drugs.
Industry: Utilized in the synthesis of chiral ligands and catalysts .
Mechanism of Action
The mechanism of action of L-tert-Leucine involves its incorporation into chiral molecules, thereby influencing their stereochemistry and biological activity. The molecular targets and pathways vary depending on the specific application, but generally, it acts by enhancing the enantioselectivity of the reactions it is involved in .
Comparison with Similar Compounds
L-Leucine: A proteinogenic amino acid with a similar structure but different applications.
L-Valine: Another branched-chain amino acid with distinct properties.
L-Isoleucine: Similar in structure but differs in the position of the methyl group
Uniqueness: L-tert-Leucine is unique due to its bulky tert-butyl side chain, which imparts distinct hydrophobic properties and steric hindrance, making it particularly useful in asymmetric synthesis and as a chiral intermediate in drug production .
Properties
IUPAC Name |
(2S)-2-amino-3,3-dimethylbutanoic acid | |
---|---|---|
Source | PubChem | |
URL | https://pubchem.ncbi.nlm.nih.gov | |
Description | Data deposited in or computed by PubChem | |
InChI |
InChI=1S/C6H13NO2/c1-6(2,3)4(7)5(8)9/h4H,7H2,1-3H3,(H,8,9)/t4-/m1/s1 | |
Source | PubChem | |
URL | https://pubchem.ncbi.nlm.nih.gov | |
Description | Data deposited in or computed by PubChem | |
InChI Key |
NPDBDJFLKKQMCM-SCSAIBSYSA-N | |
Source | PubChem | |
URL | https://pubchem.ncbi.nlm.nih.gov | |
Description | Data deposited in or computed by PubChem | |
Canonical SMILES |
CC(C)(C)C(C(=O)O)N | |
Source | PubChem | |
URL | https://pubchem.ncbi.nlm.nih.gov | |
Description | Data deposited in or computed by PubChem | |
Isomeric SMILES |
CC(C)(C)[C@@H](C(=O)O)N | |
Source | PubChem | |
URL | https://pubchem.ncbi.nlm.nih.gov | |
Description | Data deposited in or computed by PubChem | |
Molecular Formula |
C6H13NO2 | |
Source | PubChem | |
URL | https://pubchem.ncbi.nlm.nih.gov | |
Description | Data deposited in or computed by PubChem | |
DSSTOX Substance ID |
DTXSID001017204 | |
Record name | 3-Methyl-L-valine | |
Source | EPA DSSTox | |
URL | https://comptox.epa.gov/dashboard/DTXSID001017204 | |
Description | DSSTox provides a high quality public chemistry resource for supporting improved predictive toxicology. | |
Molecular Weight |
131.17 g/mol | |
Source | PubChem | |
URL | https://pubchem.ncbi.nlm.nih.gov | |
Description | Data deposited in or computed by PubChem | |
CAS No. |
20859-02-3 | |
Record name | L-tert-Leucine | |
Source | CAS Common Chemistry | |
URL | https://commonchemistry.cas.org/detail?cas_rn=20859-02-3 | |
Description | CAS Common Chemistry is an open community resource for accessing chemical information. Nearly 500,000 chemical substances from CAS REGISTRY cover areas of community interest, including common and frequently regulated chemicals, and those relevant to high school and undergraduate chemistry classes. This chemical information, curated by our expert scientists, is provided in alignment with our mission as a division of the American Chemical Society. | |
Explanation | The data from CAS Common Chemistry is provided under a CC-BY-NC 4.0 license, unless otherwise stated. | |
Record name | 3-Methyl-L-valine | |
Source | ChemIDplus | |
URL | https://pubchem.ncbi.nlm.nih.gov/substance/?source=chemidplus&sourceid=0020859023 | |
Description | ChemIDplus is a free, web search system that provides access to the structure and nomenclature authority files used for the identification of chemical substances cited in National Library of Medicine (NLM) databases, including the TOXNET system. | |
Record name | 3-Methyl-L-valine | |
Source | EPA DSSTox | |
URL | https://comptox.epa.gov/dashboard/DTXSID001017204 | |
Description | DSSTox provides a high quality public chemistry resource for supporting improved predictive toxicology. | |
Record name | L-Valine, 3-methyl | |
Source | European Chemicals Agency (ECHA) | |
URL | https://echa.europa.eu/substance-information/-/substanceinfo/100.109.056 | |
Description | The European Chemicals Agency (ECHA) is an agency of the European Union which is the driving force among regulatory authorities in implementing the EU's groundbreaking chemicals legislation for the benefit of human health and the environment as well as for innovation and competitiveness. | |
Explanation | Use of the information, documents and data from the ECHA website is subject to the terms and conditions of this Legal Notice, and subject to other binding limitations provided for under applicable law, the information, documents and data made available on the ECHA website may be reproduced, distributed and/or used, totally or in part, for non-commercial purposes provided that ECHA is acknowledged as the source: "Source: European Chemicals Agency, http://echa.europa.eu/". Such acknowledgement must be included in each copy of the material. ECHA permits and encourages organisations and individuals to create links to the ECHA website under the following cumulative conditions: Links can only be made to webpages that provide a link to the Legal Notice page. | |
Record name | 3-METHYL-L-VALINE | |
Source | FDA Global Substance Registration System (GSRS) | |
URL | https://gsrs.ncats.nih.gov/ginas/app/beta/substances/1E6RFC9IVP | |
Description | The FDA Global Substance Registration System (GSRS) enables the efficient and accurate exchange of information on what substances are in regulated products. Instead of relying on names, which vary across regulatory domains, countries, and regions, the GSRS knowledge base makes it possible for substances to be defined by standardized, scientific descriptions. | |
Explanation | Unless otherwise noted, the contents of the FDA website (www.fda.gov), both text and graphics, are not copyrighted. They are in the public domain and may be republished, reprinted and otherwise used freely by anyone without the need to obtain permission from FDA. Credit to the U.S. Food and Drug Administration as the source is appreciated but not required. | |
Retrosynthesis Analysis
AI-Powered Synthesis Planning: Our tool employs the Template_relevance Pistachio, Template_relevance Bkms_metabolic, Template_relevance Pistachio_ringbreaker, Template_relevance Reaxys, Template_relevance Reaxys_biocatalysis model, leveraging a vast database of chemical reactions to predict feasible synthetic routes.
One-Step Synthesis Focus: Specifically designed for one-step synthesis, it provides concise and direct routes for your target compounds, streamlining the synthesis process.
Accurate Predictions: Utilizing the extensive PISTACHIO, BKMS_METABOLIC, PISTACHIO_RINGBREAKER, REAXYS, REAXYS_BIOCATALYSIS database, our tool offers high-accuracy predictions, reflecting the latest in chemical research and data.
Strategy Settings
Precursor scoring | Relevance Heuristic |
---|---|
Min. plausibility | 0.01 |
Model | Template_relevance |
Template Set | Pistachio/Bkms_metabolic/Pistachio_ringbreaker/Reaxys/Reaxys_biocatalysis |
Top-N result to add to graph | 6 |
Feasible Synthetic Routes
Q1: What is the molecular formula and weight of L-tert-Leucine?
A1: this compound has a molecular formula of C6H13NO2 and a molecular weight of 131.18 g/mol.
Q2: Is there any spectroscopic data available for this compound?
A2: While the provided research articles primarily focus on the synthesis and application of this compound, spectroscopic data can be found in databases like the Human Metabolome Database (HMDB) and PubChem.
Q3: What is the role of Leucine dehydrogenase (LeuDH) in this compound production?
A3: Leucine dehydrogenase (LeuDH) is a crucial enzyme for the biocatalytic production of this compound. It catalyzes the asymmetric reduction of trimethylpyruvic acid (TMP) to this compound. [, , , ]
Q4: How can the efficiency of this compound production be improved using a coupled enzyme system?
A4: Coupling LeuDH with glucose dehydrogenase (GDH) enables efficient cofactor (NADH) regeneration. GDH oxidizes glucose to gluconic acid, simultaneously reducing NAD+ to NADH, which is then utilized by LeuDH for this compound synthesis. [, , , ]
Q5: What alternative strategies are explored for efficient cofactor regeneration in this compound biosynthesis?
A5: Apart from GDH, formate dehydrogenase (FDH) has been successfully employed in conjunction with LeuDH for the biosynthesis of this compound. FDH utilizes formate as a co-substrate for NADH regeneration. [, ]
Q6: Can whole-cell biocatalysis be applied for this compound production?
A6: Yes, genetically engineered Escherichia coli strains co-expressing LeuDH and a suitable NADH-regenerating enzyme (e.g., GDH or FDH) have been successfully used as whole-cell biocatalysts for this compound production. [, , , , , ]
Q7: Are there any challenges associated with high substrate concentrations in this compound bioproduction?
A7: High concentrations of trimethylpyruvic acid (TMP), the substrate for this compound biosynthesis, can inhibit LeuDH activity. To circumvent this, fed-batch feeding strategies have been implemented, gradually supplying TMP to the reaction system. [, , ]
Q8: Beyond this compound, what other applications do LeuDH enzymes possess?
A8: LeuDH enzymes demonstrate broad substrate specificity, showing activity towards various L-amino acids and α-keto acids. This versatility allows their application in the synthesis of other non-natural amino acids, expanding their utility in pharmaceutical and biotechnological applications. [, ]
Q9: What is the impact of salt and organic solvents on the activity of PtxD enzymes used for NADH regeneration?
A9: Phosphite dehydrogenase (PtxD) from Cyanothece sp. ATCC 51142 (Ct-PtxD) shows remarkable tolerance to salt ions and organic solvents compared to PtxD from other organisms. This enhanced stability makes Ct-PtxD a promising candidate for industrial NADH regeneration applications. []
Q10: How does temperature affect the activity of LeuDH enzymes?
A10: LeuDH enzymes exhibit varying temperature optima. Some LeuDHs, like those derived from Exiguobacterium sibiricum and Pseudomonas balearica, exhibit high thermostability, while others, like those from Antarctic bacteria, display optimal activity at lower temperatures. [, , ]
Q11: How does the N-terminal protecting group on aza-dipeptide HIV protease inhibitors affect their oral bioavailability?
A11: Studies on aza-dipeptide HIV protease inhibitors reveal that incorporating an N-(methoxycarbonyl)-L-tert-leucinyl moiety as the nitrogen protecting group significantly enhances oral bioavailability compared to L-valine or L-isoleucine derivatives. This highlights the impact of steric bulk and chirality on pharmacokinetic properties. [, ]
Q12: How do structural modifications on chiral tertiary amine-urea catalysts impact their chemoselectivity in reactions involving 5H-oxazol-4-ones and N-itaconimides?
A12: Modifying the steric and electronic properties of the this compound-derived tertiary amine-urea catalysts can switch the chemoselectivity of the reaction between 5H-oxazol-4-ones and N-itaconimides. Subtle changes to the catalyst structure can favor either tandem conjugate addition-protonation or [4+2] cycloaddition pathways. [, ]
Q13: What are some valuable resources for discovering novel enzymes involved in this compound synthesis?
A13: Genome mining has proven to be a powerful tool for identifying novel LeuDH enzymes with desirable properties. Bioinformatics databases and tools are instrumental in analyzing gene sequences, predicting protein structures, and exploring potential enzymatic activities. [, , ]
Disclaimer and Information on In-Vitro Research Products
Please be aware that all articles and product information presented on BenchChem are intended solely for informational purposes. The products available for purchase on BenchChem are specifically designed for in-vitro studies, which are conducted outside of living organisms. In-vitro studies, derived from the Latin term "in glass," involve experiments performed in controlled laboratory settings using cells or tissues. It is important to note that these products are not categorized as medicines or drugs, and they have not received approval from the FDA for the prevention, treatment, or cure of any medical condition, ailment, or disease. We must emphasize that any form of bodily introduction of these products into humans or animals is strictly prohibited by law. It is essential to adhere to these guidelines to ensure compliance with legal and ethical standards in research and experimentation.