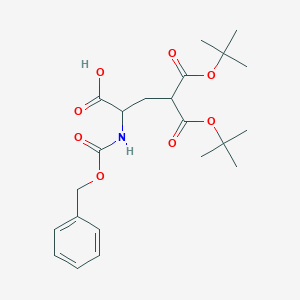
Z-DL-Gla(OtBu)2-OH
Overview
Description
Z-DL-Gla(OtBu)2-OH is a chemical compound with the molecular formula C22H31NO8 and a molecular weight of 437.48 . It is a building block for the introduction of γ-carboxyglutamic acid (Gla). γ-carboxylation of glutamic acid is a rare post-translational modification that occurs in blood coagulation factors and in some snake and cone snail venoms .
Molecular Structure Analysis
The molecular structure of Z-DL-Gla(OtBu)2-OH includes 62 bonds in total, with 31 non-H bonds, 10 multiple bonds, 13 rotatable bonds, 4 double bonds, 6 aromatic bonds, 1 six-membered ring, 1 carboxylic acid (aliphatic), 2 esters (aliphatic), and 1 (thio-) carbamate (aliphatic) .Physical And Chemical Properties Analysis
Z-DL-Gla(OtBu)2-OH has a predicted boiling point of 582.6±50.0 °C and a predicted density of 1.188±0.06 g/cm3 . The pKa is predicted to be 3.59±0.10 . The storage temperature is -15°C .Scientific Research Applications
Blood Coagulation
“Z-DL-Gla(OtBu)2-OH” is a building block for the introduction of γ-carboxyglutamic acid (Gla), which plays a crucial role in blood coagulation. Gla residues are essential for the high-affinity calcium binding sites in the GLA domain of coagulation factors, such as factor IX, a serine protease of the coagulation system. These sites mediate the binding of factor IXa to platelets and are involved in factor-X activation .
Vitamin K-dependent Proteins
Vitamin K acts as a cofactor in the enzymatic conversion of glutamic acid residues to γ-carboxyglutamic acid (Gla) in vitamin K-dependent proteins. This process is catalyzed by the enzyme γ-glutamyl carboxylase located in the endoplasmic reticulum .
Membrane Anchors for Clotting Factors
Gla-rich modules were traditionally viewed as membrane anchors that enable vitamin K–dependent clotting factors to interact with negatively charged phospholipid surfaces, where they can effectively exert their pro- and anticoagulant activities .
Bone Matrix Formation
The gamma-carboxyglutamic acid-rich (GLA) domain is abundant in proteins involved in bone matrix formation, indicating a significant role for Gla in this field .
Enzyme-Cofactor Complexes
On phospholipid surfaces, Gla domains facilitate the interaction of enzyme-cofactor complexes that are vital for their pro- and anticoagulant functions .
Proteomics Research
“Z-DL-Gla(OtBu)2-OH” is used as a specialty product for proteomics research, aiding in the investigation and identification of protein γ-glutamyl carboxylation .
Safety and Hazards
properties
IUPAC Name |
5-[(2-methylpropan-2-yl)oxy]-4-[(2-methylpropan-2-yl)oxycarbonyl]-5-oxo-2-(phenylmethoxycarbonylamino)pentanoic acid | |
---|---|---|
Source | PubChem | |
URL | https://pubchem.ncbi.nlm.nih.gov | |
Description | Data deposited in or computed by PubChem | |
InChI |
InChI=1S/C22H31NO8/c1-21(2,3)30-18(26)15(19(27)31-22(4,5)6)12-16(17(24)25)23-20(28)29-13-14-10-8-7-9-11-14/h7-11,15-16H,12-13H2,1-6H3,(H,23,28)(H,24,25) | |
Source | PubChem | |
URL | https://pubchem.ncbi.nlm.nih.gov | |
Description | Data deposited in or computed by PubChem | |
InChI Key |
JSRFPOKYPNCYJU-UHFFFAOYSA-N | |
Source | PubChem | |
URL | https://pubchem.ncbi.nlm.nih.gov | |
Description | Data deposited in or computed by PubChem | |
Canonical SMILES |
CC(C)(C)OC(=O)C(CC(C(=O)O)NC(=O)OCC1=CC=CC=C1)C(=O)OC(C)(C)C | |
Source | PubChem | |
URL | https://pubchem.ncbi.nlm.nih.gov | |
Description | Data deposited in or computed by PubChem | |
Molecular Formula |
C22H31NO8 | |
Source | PubChem | |
URL | https://pubchem.ncbi.nlm.nih.gov | |
Description | Data deposited in or computed by PubChem | |
DSSTOX Substance ID |
DTXSID10452839 | |
Record name | 2-{[(Benzyloxy)carbonyl]amino}-5-tert-butoxy-4-(tert-butoxycarbonyl)-5-oxopentanoic acid (non-preferred name) | |
Source | EPA DSSTox | |
URL | https://comptox.epa.gov/dashboard/DTXSID10452839 | |
Description | DSSTox provides a high quality public chemistry resource for supporting improved predictive toxicology. | |
Molecular Weight |
437.5 g/mol | |
Source | PubChem | |
URL | https://pubchem.ncbi.nlm.nih.gov | |
Description | Data deposited in or computed by PubChem | |
Product Name |
Z-DL-Gla(OtBu)2-OH | |
CAS RN |
56877-43-1 | |
Record name | 1,1-Bis(1,1-dimethylethyl) 3-[[(phenylmethoxy)carbonyl]amino]-1,1,3-propanetricarboxylate | |
Source | CAS Common Chemistry | |
URL | https://commonchemistry.cas.org/detail?cas_rn=56877-43-1 | |
Description | CAS Common Chemistry is an open community resource for accessing chemical information. Nearly 500,000 chemical substances from CAS REGISTRY cover areas of community interest, including common and frequently regulated chemicals, and those relevant to high school and undergraduate chemistry classes. This chemical information, curated by our expert scientists, is provided in alignment with our mission as a division of the American Chemical Society. | |
Explanation | The data from CAS Common Chemistry is provided under a CC-BY-NC 4.0 license, unless otherwise stated. | |
Record name | 2-{[(Benzyloxy)carbonyl]amino}-5-tert-butoxy-4-(tert-butoxycarbonyl)-5-oxopentanoic acid (non-preferred name) | |
Source | EPA DSSTox | |
URL | https://comptox.epa.gov/dashboard/DTXSID10452839 | |
Description | DSSTox provides a high quality public chemistry resource for supporting improved predictive toxicology. | |
Retrosynthesis Analysis
AI-Powered Synthesis Planning: Our tool employs the Template_relevance Pistachio, Template_relevance Bkms_metabolic, Template_relevance Pistachio_ringbreaker, Template_relevance Reaxys, Template_relevance Reaxys_biocatalysis model, leveraging a vast database of chemical reactions to predict feasible synthetic routes.
One-Step Synthesis Focus: Specifically designed for one-step synthesis, it provides concise and direct routes for your target compounds, streamlining the synthesis process.
Accurate Predictions: Utilizing the extensive PISTACHIO, BKMS_METABOLIC, PISTACHIO_RINGBREAKER, REAXYS, REAXYS_BIOCATALYSIS database, our tool offers high-accuracy predictions, reflecting the latest in chemical research and data.
Strategy Settings
Precursor scoring | Relevance Heuristic |
---|---|
Min. plausibility | 0.01 |
Model | Template_relevance |
Template Set | Pistachio/Bkms_metabolic/Pistachio_ringbreaker/Reaxys/Reaxys_biocatalysis |
Top-N result to add to graph | 6 |
Feasible Synthetic Routes
Disclaimer and Information on In-Vitro Research Products
Please be aware that all articles and product information presented on BenchChem are intended solely for informational purposes. The products available for purchase on BenchChem are specifically designed for in-vitro studies, which are conducted outside of living organisms. In-vitro studies, derived from the Latin term "in glass," involve experiments performed in controlled laboratory settings using cells or tissues. It is important to note that these products are not categorized as medicines or drugs, and they have not received approval from the FDA for the prevention, treatment, or cure of any medical condition, ailment, or disease. We must emphasize that any form of bodily introduction of these products into humans or animals is strictly prohibited by law. It is essential to adhere to these guidelines to ensure compliance with legal and ethical standards in research and experimentation.