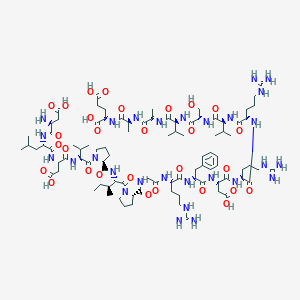
Calcineurin substrate
Overview
Description
Calcineurin (CaN), a calcium/calmodulin-dependent serine/threonine protein phosphatase, is a heterodimer comprising a catalytic subunit (CaNA) and a regulatory subunit (CaNB) . It plays critical roles in immune response, cardiac development, neuronal signaling, and fungal pathogenesis. Calcineurin substrates are phosphorylated proteins that undergo dephosphorylation upon CaN activation, triggering downstream signaling cascades. Key substrates include transcription factors (e.g., NFAT, Crz1), structural proteins (e.g., POC5), and enzymes (e.g., PDE5) .
Calcineurin activity is assayed using synthetic phosphopeptides like the RII phosphopeptide (DLDVPIPGRFDRRVSVAAE), a high-affinity substrate with a CAS number 113873-67-9 and molecular weight 2112.35 g/mol . This substrate is widely used to study CaN kinetics and inhibition .
Preparation Methods
Calcineurin substrates are typically synthesized using standard peptide synthesis techniques. The synthetic routes involve the stepwise addition of amino acids to a growing peptide chain, using solid-phase peptide synthesis (SPPS) or liquid-phase peptide synthesis (LPPS). The reaction conditions often include the use of protecting groups to prevent unwanted side reactions and the use of coupling reagents to facilitate peptide bond formation .
In industrial production, calcineurin substrates are produced using recombinant DNA technology. This involves the insertion of the gene encoding the substrate into a suitable expression vector, which is then introduced into a host organism such as Escherichia coli or yeast. The host organism expresses the substrate, which is subsequently purified using chromatographic techniques .
Chemical Reactions Analysis
Catalytic Mechanism for pNPP Hydrolysis
The hydrolysis of p-nitrophenyl phosphate (pNPP) serves as a model reaction for studying calcineurin's phosphoryl-transfer mechanism. Heavy-atom isotope effect measurements reveal:
Isotope Effect | pH 7.0 (1 mM Mn²⁺) | pH 8.5 (5 mM Mn²⁺) | Mechanistic Implication |
---|---|---|---|
¹⁸(V/K)_bridge | 1.0072 | 1.0115 | P-O bond cleavage rate |
¹⁵(V/K) | 1.0006 | 1.0014 | Leaving group stability |
¹⁸(V/K)_nonbridge | - | 0.9942 | Dianion substrate form |
These data indicate a dissociative transition state with partial P-O bond cleavage at physiological pH (7.0) that becomes more rate-limiting under alkaline conditions (8.5) . Mn²⁺ concentration increases mimic pH effects by reducing commitment factors, making the chemical step more kinetically significant .
Substrate Recognition Motifs
Calcineurin engages substrates through two conserved binding regions:
PxIxIT motif
-
Binds hydrophobic groove (β11-β14 sheets)
-
Maintains binding regardless of enzyme activation state
ΦLxVP motif
-
Targets substrate-binding cleft near catalytic site
-
Binding affinity modulated by activation state:
Mutant | Kᴅ (nM) | ΔG (kcal/mol) | Kᵢ (nM) |
---|---|---|---|
Wild-type A238L | 4 ± 1 | -11.5 ± 0.2 | 0.37 |
PxIxIT mutant | 624 ± 26 | -8.5 ± 0.0 | 15 |
ΦLxVP mutant (FLCVK) | 803 ± 26 | -8.3 ± 0.0 | 7,700 |
ΦLxVP interactions contribute >90% of binding energy, making them essential for catalytic engagement .
Activation-Dependent Conformational Changes
Calcineurin's catalytic efficiency depends on sequential structural modifications:
-
Ca²⁺ binding to calcineurin B's EF-hands (Kᴅ: 1-2 μM for sites 1-2, nM for 3-4)
-
Calmodulin binding displaces autoinhibitory domain (AID) from catalytic site
-
Substrate docking via PxIxIT/ΦLxVP motifs enables precise active-site alignment
Activation increases V_max for pNPP hydrolysis by 400-600% while reducing Kₘ 3-fold, indicating both enhanced catalytic rate and substrate affinity .
Metal Cofactor Requirements
The enzyme's binuclear metal center requires:
-
Fe³⁺ in the high-affinity site (Kᴅ ≈ 10⁻¹⁸ M)
-
Zn²⁺ in the low-affinity site (Kᴅ ≈ 10⁻⁹ M)
-
Mn²⁺/Mg²⁺ as exchangeable activators (Kᴅ ≈ 10⁻⁶ M)
Metal substitution experiments show Mn²⁺ > Mg²⁺ > Ca²⁺ for catalytic activation, with k_cat/Kₘ varying 100-fold across divalent cations .
pH-Dependent Activity Profile
Calcineurin exhibits optimal activity at pH 7.0-7.5 with:
-
pKₐ1 = 6.2 ± 0.1 (histidine protonation)
-
pKₐ2 = 7.8 ± 0.2 (catalytic base activation)
The pH-rate profile correlates with protonation states of His¹⁵¹ (substrate stabilization) and Asp⁹² (nucleophilic water activation) in the active site . At pH >8.0, rate limitation shifts from metal-hydroxide formation to P-O bond cleavage .
These biochemical and structural insights establish calcineurin as a metallophosphatase with sophisticated substrate discrimination mechanisms, making it a critical regulatory node in calcium-dependent signaling pathways.
Scientific Research Applications
Key Applications
-
Immunosuppressive Therapy
- Calcineurin Inhibitors : Drugs such as cyclosporine and tacrolimus inhibit calcineurin activity, which is pivotal in preventing organ transplant rejection by inhibiting T-cell activation. These inhibitors block the transcription of interleukin-2 and other cytokines essential for T-cell proliferation .
- Clinical Indications : Calcineurin inhibitors are used to manage autoimmune diseases like lupus nephritis and rheumatoid arthritis, as well as in solid organ transplantation protocols .
-
Fungal Pathogenesis
- Role in Virulence : In pathogenic fungi like Cryptococcus neoformans, calcineurin substrates are involved in stress survival and virulence. Recent studies have identified novel calcineurin targets that regulate mRNA metabolism and contribute to fungal pathogenicity under stress conditions .
- Potential Therapeutic Targets : The identification of calcineurin substrates involved in fungal virulence presents opportunities for developing antifungal therapies targeting these pathways .
-
Neurobiology
- Calcium Signaling : Calcineurin substrates play a role in neuronal signaling pathways, affecting synaptic plasticity and memory formation. Dysregulation of this pathway has implications for neurodegenerative diseases .
- Toxic Responses : Research indicates that certain proteins can modulate calcineurin activity, leading to either protective or toxic responses in neurons depending on the context of calcium signaling .
Case Study 1: Calcineurin in Fungal Virulence
A study utilizing phosphoproteomic techniques identified several calcineurin substrates in Cryptococcus neoformans. These substrates were linked to stress granule formation and mRNA regulation, highlighting their role in the organism's survival under adverse conditions. The study emphasized the potential for targeting these pathways to develop new antifungal treatments .
Case Study 2: Immunosuppressive Drug Mechanisms
Research into the mechanisms of action of calcineurin inhibitors revealed that these drugs bind to immunophilins, forming complexes that inhibit calcineurin's phosphatase activity. This inhibition prevents the activation of nuclear factor of activated T cells (NFAT), thereby reducing cytokine production necessary for T-cell activation . This understanding has led to optimized therapeutic regimens for transplant patients.
Data Table: Summary of Calcineurin Substrates
Substrate Name | Function | Application Area | Notes |
---|---|---|---|
Crz1 | Transcriptional regulation | Fungal virulence | Essential for stress response in C. neoformans |
NFAT | Cytokine gene transcription | Immunology | Targeted by calcineurin inhibitors |
Pbp1 | mRNA metabolism | Fungal virulence | Regulates stress granules during thermal stress |
Puf4 | RNA binding | Fungal virulence | Involved in post-transcriptional regulation |
Mechanism of Action
The mechanism of action of calcineurin substrates involves their dephosphorylation by calcineurin. Calcineurin is activated by the binding of calcium ions and calmodulin, which induces a conformational change that allows the enzyme to interact with its substrates . The dephosphorylation of the substrate leads to changes in its activity, stability, or localization, which in turn affects downstream signaling pathways . Molecular targets of calcineurin substrates include transcription factors such as nuclear factor of activated T-cells (NFAT), which play a key role in immune responses .
Comparison with Similar Compounds
Substrate Affinity and Dephosphorylation Efficiency
Calcineurin exhibits substrate preference based on structural motifs and binding affinity:
- Crz1 : A high-affinity substrate in yeast, rapidly dephosphorylated by CaN (Fig. 2A). Dephosphorylation is more efficient compared to Aly1, where only a subset of phospho-sites are targeted .
- Aly1 : Partial dephosphorylation by CaN, suggesting lower affinity or steric hindrance. γ-phosphatase fully dephosphorylates Aly1, highlighting CaN's substrate specificity .
- RII Phosphopeptide : A synthetic substrate with an IC₅₀ of 3.5 ± 0.4 μM for CaN inhibition by CN585 . Kinetic studies reveal reversible inhibition (Ki = 3.8 ± 0.3 μM) .
- POC5 : Contains a PxIxIT motif that mediates CaN binding. CaN colocalizes with POC5 at centrioles, regulating cilia length. Inhibition alters POC5 distribution, emphasizing motif-dependent interactions .
Table 1: Substrate-Specific Kinetic Parameters
*Estimated based on comparative kinetics .
Inhibitors and Their Selectivity
Calcineurin inhibitors vary in mechanism, potency, and specificity:
- CN585: A reversible inhibitor with Ki = 3.8 μM and IC₅₀ = 3.5 μM against RII phosphopeptide. Exhibits non-competitive inhibition, as shown by intersecting lines in Dixon plots .
- Irregularasulfate 209/Hipposulfate C210: Natural compounds with IC₅₀ values of 59–69 μM against CaN. These sulfated terpenoids also inhibit PP-1 (IC₅₀ = 64–71 μM) but are less active against PP-2A (IC₅₀ = 130–140 μM), suggesting partial selectivity .
- FK506/Cyclosporine A (CsA): Immunosuppressants forming complexes with immunophilins to inhibit CaN. Both prevent mitochondrial dysfunction in neurons but differ in secondary targets (e.g., CsA affects mPTP opening) .
- Fungal-Specific Inhibitors : Designed to exploit structural differences between fungal and human CaN. For example, LQLP motif-targeting compounds show species-specific efficacy .
Table 2: Inhibitor Profiles
*Phosphate analogue of halisulfate-7 .
Structural and Mechanistic Insights
- Motif Recognition :
- Post-Translational Modifications: Myristoylation of CaNB influences substrate preference. Non-myristoylated CaN (Cnb1-G2A) shows altered Crz1 dephosphorylation and increased FK506 sensitivity .
- Catalytic Mechanism: CaN’s active site shares homology with acid sphingomyelinase (ASMase) but differs in proton donor residues (His280 in ASMase vs. Arg in CaN) .
Biological Activity
Calcineurin, a calcium-activated protein phosphatase, plays a crucial role in various biological processes by dephosphorylating target proteins, thereby modulating their activity. This article delves into the biological activity of calcineurin substrates, highlighting their mechanisms of action, interactions, and implications in health and disease.
Overview of Calcineurin Function
Calcineurin consists of two subunits: a catalytic A subunit and a regulatory B subunit. It is activated by calcium ions binding to calmodulin, which then associates with the catalytic subunit, facilitating its enzymatic activity. The primary function of calcineurin is to dephosphorylate serine and threonine residues on target proteins, particularly the nuclear factor of activated T cells (NFAT) family of transcription factors, which are pivotal in immune response regulation .
The activation of calcineurin leads to a cascade of signaling events:
- Calcium Influx : Increased intracellular calcium levels activate calmodulin.
- Calcineurin Activation : The calcium-calmodulin complex binds to calcineurin A, triggering its activation.
- Dephosphorylation : Calcineurin dephosphorylates NFAT, causing it to undergo a conformational change that exposes its nuclear localization sequence.
- Nuclear Translocation : NFAT translocates to the nucleus and activates transcription of genes involved in T-cell activation and other physiological processes .
Key Substrates and Their Roles
Calcineurin interacts with a variety of substrates, each playing specific roles in cellular signaling and function. Some notable substrates include:
Substrate | Function | Biological Significance |
---|---|---|
NFAT | Transcription factor | Regulates immune response and development |
Crz1 | Transcription factor | Involved in stress response in fungi |
Pbp1 | mRNA-binding protein | Regulates mRNA stability and translation |
Puf4 | RNA-binding protein | Modulates gene expression post-transcriptionally |
These substrates illustrate the diverse roles calcineurin plays beyond immune signaling, including stress responses in fungi and mRNA metabolism .
1. Calcineurin in Fungal Virulence
A study on Cryptococcus neoformans identified calcineurin substrates involved in stress survival and virulence. Researchers utilized phosphoproteomic techniques to characterize targets such as Crz1, which governs transcriptional responses under stress conditions. This study revealed that calcineurin not only regulates transcription but also influences post-transcriptional processes by interacting with proteins involved in mRNA metabolism .
2. Calcineurin's Role in Cardiac Function
Research has shown that calcineurin is critical for cardiac hypertrophy and remodeling. In models of heart disease, increased calcineurin activity leads to enhanced NFAT signaling, contributing to pathological cardiac growth. Targeting this pathway has therapeutic potential for preventing heart failure .
3. Implications in Autoimmune Diseases
Calcineurin inhibitors like cyclosporin A and FK506 are used clinically to prevent organ transplant rejection by inhibiting T-cell activation through blocking calcineurin's action on NFAT. Understanding the substrate interactions provides insights into developing more selective inhibitors with fewer side effects .
Q & A
Q. (Basic) What experimental methodologies are most reliable for identifying novel calcineurin substrates in cellular contexts?
To identify novel substrates, combine phosphatase activity assays (using substrates like RII phosphopeptide ) with phosphoproteomic screens under calcium/calmodulin stimulation. Key steps:
In vitro dephosphorylation assays : Use recombinant calcineurin (e.g., human heterodimer Aα/B) with candidate peptides under Ca²⁺/calmodulin activation .
Mass spectrometry (MS)-based phosphoproteomics : Treat cells with calcineurin inhibitors (e.g., cyclosporin A) to stabilize phosphorylated substrates, then compare phosphorylation sites with controls .
Mutagenesis validation : Replace predicted calcineurin-binding motifs (e.g., PxIxIT in NFAT) and assess phosphatase susceptibility .
Table 1 : Common calcineurin substrates and their validation methods
Q. (Advanced) How can conflicting data on calcineurin substrate specificity be systematically resolved?
Conflicts often arise from divergent assay conditions (e.g., Ca²⁺ concentration, calmodulin purity) or cell-type-specific regulatory factors . To address this:
Standardize assay buffers : Use 1 µM free Ca²⁺ and 100 nM calmodulin to mimic physiological conditions .
Cross-validate findings : Combine in vitro assays (e.g., surface plasmon resonance for binding affinity) with in-cell western blotting .
Control for endogenous inhibitors (e.g., Cabin1): Use siRNA knockdown or inhibitor-free lysates .
Example conflict : Discrepancies in AKAP79 binding may stem from calmodulin competition. Resolve via FRET-based competition assays .
Q. (Basic) What computational tools are effective for predicting calcineurin-substrate interactions?
Leverage generative machine learning models trained on known binding motifs (e.g., PxIxIT, LxVP). Tools include:
AlphaFold-Multimer : Predicts 3D structures of calcineurin-substrate complexes .
PepGAN : Generates peptide sequences mimicking natural substrates, validated via molecular docking .
Motif enrichment analysis : Use databases like ELM (Eukaryotic Linear Motif) to identify conserved binding regions .
Q. (Advanced) How to design in vivo studies to capture dynamic calcineurin-substrate interactions?
Use conditional knockout models : Tissue-specific calcineurin deletion (e.g., Cre-lox in neurons) paired with phospho-specific antibodies .
Real-time imaging : FRET biosensors (e.g., NFAT-EGFP) to monitor substrate dephosphorylation kinetics .
Transcriptional reporters : Luciferase-coupled NFAT response elements to quantify calcineurin activity post-stimulation .
Key challenge : Temporal resolution. Address via optogenetic calcineurin activation paired with rapid sampling .
Q. (Advanced) What strategies validate the physiological relevance of putative substrates identified in vitro?
Knockdown/rescue experiments : Silence the substrate and reintroduce wild-type/mutant versions to assess calcineurin-dependent phenotypes (e.g., T-cell activation for NFAT) .
In vivo crosslinking : Use formaldehyde fixation to stabilize transient interactions, followed by co-IP/MS .
Pathway enrichment analysis : Link substrates to calcineurin-regulated processes (e.g., immune response, cardiac hypertrophy) via RNA-seq .
Q. (Basic) How to optimize calcineurin activity assays for high-throughput screening (HTS)?
Substrate choice : Use synthetic peptides (e.g., [pSer95]-RII) with high solubility and low non-specific binding .
Detection method : Colorimetric assays (e.g., malachite green for phosphate release) compatible with 384-well plates .
Control wells : Include calmodulin-free and cyclosporin A-treated conditions to confirm calcineurin-specific activity .
Table 2 : HTS parameters for calcineurin assays
Parameter | Optimal Condition |
---|---|
Temperature | 30°C |
Reaction Time | 30 min |
Ca²⁺ Concentration | 1 µM |
Signal Threshold | ≥3x background |
Q. (Advanced) What experimental and statistical approaches address low reproducibility in substrate kinetic studies?
Precision-recall analysis : Quantify assay sensitivity/specificity using ROC curves .
Replicate stratification : Run technical replicates across multiple plates and biological replicates from independent cell cultures .
Data normalization : Use internal standards (e.g., non-hydrolyzable ATP) to control for plate-to-plate variability .
Q. (Basic) How to distinguish calcineurin-specific dephosphorylation from other phosphatases (e.g., PP1/PP2A)?
Pharmacological inhibition : Pre-treat samples with 1 µM cyclosporin A (calcineurin-specific) vs. okadaic acid (PP1/PP2A inhibitor) .
Substrate competition : Add excess PxIxIT peptide to block calcineurin binding .
Genetic ablation : Compare substrate dephosphorylation in wild-type vs. calcineurin-deficient cells .
Q. (Advanced) What emerging technologies enhance resolution in calcineurin-substrate interaction studies?
Cryo-EM : Resolve calcineurin-substrate complexes at near-atomic resolution (≤3 Å) .
Single-molecule fluorescence : Track real-time binding/dissociation kinetics using TIRF microscopy .
Proximity labeling : TurboID-fused calcineurin to biotinylate proximal substrates for MS identification .
Q. (Advanced) How to evaluate the functional impact of substrate mutations on calcineurin signaling networks?
Network perturbation analysis : Use CRISPR-Cas9 to introduce mutations and model downstream effects via Boolean networks .
Dynamic pathway mapping : Integrate phosphoproteomics with RNA-seq to link substrate dephosphorylation to transcriptional changes .
Phenotypic scoring : Quantify immune activation or hypertrophy markers in mutant vs. wild-type models .
Properties
IUPAC Name |
(2S)-2-[[(2S)-2-[[(2S)-2-[[(2S)-2-[[(2S)-2-[[(2S)-2-[[(2S)-2-[[(2S)-2-[[(2S)-2-[[(2S)-2-[[(2S)-2-[[2-[[(2S)-1-[(2S,3S)-2-[[(2S)-1-[(2S)-2-[[(2S)-2-[[(2S)-2-[[(2S)-2-amino-3-carboxypropanoyl]amino]-4-methylpentanoyl]amino]-3-carboxypropanoyl]amino]-3-methylbutanoyl]pyrrolidine-2-carbonyl]amino]-3-methylpentanoyl]pyrrolidine-2-carbonyl]amino]acetyl]amino]-5-carbamimidamidopentanoyl]amino]-3-phenylpropanoyl]amino]-3-carboxypropanoyl]amino]-5-carbamimidamidopentanoyl]amino]-5-carbamimidamidopentanoyl]amino]-3-methylbutanoyl]amino]-3-hydroxypropanoyl]amino]-3-methylbutanoyl]amino]propanoyl]amino]propanoyl]amino]pentanedioic acid | |
---|---|---|
Source | PubChem | |
URL | https://pubchem.ncbi.nlm.nih.gov | |
Description | Data deposited in or computed by PubChem | |
InChI |
InChI=1S/C92H150N28O29/c1-13-47(10)71(118-84(143)62-28-21-35-120(62)87(146)70(46(8)9)117-81(140)59(40-67(129)130)113-78(137)56(36-43(2)3)110-74(133)51(93)38-65(125)126)88(147)119-34-20-27-61(119)83(142)103-41-63(122)106-52(24-17-31-100-90(94)95)75(134)111-57(37-50-22-15-14-16-23-50)79(138)112-58(39-66(127)128)80(139)108-53(25-18-32-101-91(96)97)76(135)107-54(26-19-33-102-92(98)99)77(136)115-69(45(6)7)86(145)114-60(42-121)82(141)116-68(44(4)5)85(144)105-48(11)72(131)104-49(12)73(132)109-55(89(148)149)29-30-64(123)124/h14-16,22-23,43-49,51-62,68-71,121H,13,17-21,24-42,93H2,1-12H3,(H,103,142)(H,104,131)(H,105,144)(H,106,122)(H,107,135)(H,108,139)(H,109,132)(H,110,133)(H,111,134)(H,112,138)(H,113,137)(H,114,145)(H,115,136)(H,116,141)(H,117,140)(H,118,143)(H,123,124)(H,125,126)(H,127,128)(H,129,130)(H,148,149)(H4,94,95,100)(H4,96,97,101)(H4,98,99,102)/t47-,48-,49-,51-,52-,53-,54-,55-,56-,57-,58-,59-,60-,61-,62-,68-,69-,70-,71-/m0/s1 | |
Source | PubChem | |
URL | https://pubchem.ncbi.nlm.nih.gov | |
Description | Data deposited in or computed by PubChem | |
InChI Key |
CNNRZOUCQVLOST-RBHZDPOXSA-N | |
Source | PubChem | |
URL | https://pubchem.ncbi.nlm.nih.gov | |
Description | Data deposited in or computed by PubChem | |
Canonical SMILES |
CCC(C)C(C(=O)N1CCCC1C(=O)NCC(=O)NC(CCCNC(=N)N)C(=O)NC(CC2=CC=CC=C2)C(=O)NC(CC(=O)O)C(=O)NC(CCCNC(=N)N)C(=O)NC(CCCNC(=N)N)C(=O)NC(C(C)C)C(=O)NC(CO)C(=O)NC(C(C)C)C(=O)NC(C)C(=O)NC(C)C(=O)NC(CCC(=O)O)C(=O)O)NC(=O)C3CCCN3C(=O)C(C(C)C)NC(=O)C(CC(=O)O)NC(=O)C(CC(C)C)NC(=O)C(CC(=O)O)N | |
Source | PubChem | |
URL | https://pubchem.ncbi.nlm.nih.gov | |
Description | Data deposited in or computed by PubChem | |
Isomeric SMILES |
CC[C@H](C)[C@@H](C(=O)N1CCC[C@H]1C(=O)NCC(=O)N[C@@H](CCCNC(=N)N)C(=O)N[C@@H](CC2=CC=CC=C2)C(=O)N[C@@H](CC(=O)O)C(=O)N[C@@H](CCCNC(=N)N)C(=O)N[C@@H](CCCNC(=N)N)C(=O)N[C@@H](C(C)C)C(=O)N[C@@H](CO)C(=O)N[C@@H](C(C)C)C(=O)N[C@@H](C)C(=O)N[C@@H](C)C(=O)N[C@@H](CCC(=O)O)C(=O)O)NC(=O)[C@@H]3CCCN3C(=O)[C@H](C(C)C)NC(=O)[C@H](CC(=O)O)NC(=O)[C@H](CC(C)C)NC(=O)[C@H](CC(=O)O)N | |
Source | PubChem | |
URL | https://pubchem.ncbi.nlm.nih.gov | |
Description | Data deposited in or computed by PubChem | |
Molecular Formula |
C92H150N28O29 | |
Source | PubChem | |
URL | https://pubchem.ncbi.nlm.nih.gov | |
Description | Data deposited in or computed by PubChem | |
DSSTOX Substance ID |
DTXSID20583284 | |
Record name | L-alpha-Aspartyl-L-leucyl-L-alpha-aspartyl-L-valyl-L-prolyl-L-isoleucyl-L-prolylglycyl-L-arginyl-L-phenylalanyl-L-alpha-aspartyl-L-arginyl-L-arginyl-L-valyl-L-seryl-L-valyl-L-alanyl-L-alanyl-L-glutamic acid | |
Source | EPA DSSTox | |
URL | https://comptox.epa.gov/dashboard/DTXSID20583284 | |
Description | DSSTox provides a high quality public chemistry resource for supporting improved predictive toxicology. | |
Molecular Weight |
2112.3 g/mol | |
Source | PubChem | |
URL | https://pubchem.ncbi.nlm.nih.gov | |
Description | Data deposited in or computed by PubChem | |
CAS No. |
113873-67-9 | |
Record name | L-alpha-Aspartyl-L-leucyl-L-alpha-aspartyl-L-valyl-L-prolyl-L-isoleucyl-L-prolylglycyl-L-arginyl-L-phenylalanyl-L-alpha-aspartyl-L-arginyl-L-arginyl-L-valyl-L-seryl-L-valyl-L-alanyl-L-alanyl-L-glutamic acid | |
Source | EPA DSSTox | |
URL | https://comptox.epa.gov/dashboard/DTXSID20583284 | |
Description | DSSTox provides a high quality public chemistry resource for supporting improved predictive toxicology. | |
Disclaimer and Information on In-Vitro Research Products
Please be aware that all articles and product information presented on BenchChem are intended solely for informational purposes. The products available for purchase on BenchChem are specifically designed for in-vitro studies, which are conducted outside of living organisms. In-vitro studies, derived from the Latin term "in glass," involve experiments performed in controlled laboratory settings using cells or tissues. It is important to note that these products are not categorized as medicines or drugs, and they have not received approval from the FDA for the prevention, treatment, or cure of any medical condition, ailment, or disease. We must emphasize that any form of bodily introduction of these products into humans or animals is strictly prohibited by law. It is essential to adhere to these guidelines to ensure compliance with legal and ethical standards in research and experimentation.