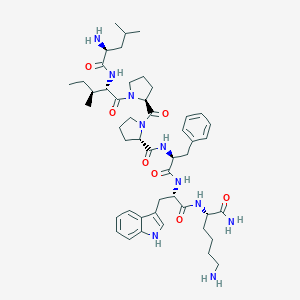
Naja mossambica mossambica alpha-neurotoxin I
Overview
Description
Naja mossambica mossambica alpha-neurotoxin I is a potent neurotoxin derived from the venom of the Mozambique spitting cobra (Naja mossambica mossambica). This compound is part of a family of alpha-neurotoxins known for their ability to bind to nicotinic acetylcholine receptors at the neuromuscular junction, leading to paralysis.
Scientific Research Applications
Naja mossambica mossambica alpha-neurotoxin I has several scientific research applications:
Neuroscience Research: It is used to study the function of nicotinic acetylcholine receptors and the mechanisms of synaptic transmission.
Drug Development: The neurotoxin serves as a lead compound for developing drugs targeting nicotinic acetylcholine receptors, which are implicated in various neurological disorders.
Toxinology: Research on this neurotoxin helps in understanding the diversity and evolution of snake venoms.
Medical Research: It is used in the development of antivenoms and in studying the pathophysiology of snakebite envenomation
Mechanism of Action
The alpha-neurotoxin from Naja mossambica venom acts on postsynaptic nicotinic acetylcholine receptors at the neuromuscular junction, leading to clinically significant skeletal muscle paralysis following envenomation . The toxin’s mechanism of action involves neuromuscular blockade, reversibility of blockade, and differential binding affinities for the two different acetylcholine-binding sites on the muscle nicotinic receptor .
Safety and Hazards
Future Directions
Research on the alpha-neurotoxin from Naja mossambica venom is ongoing, with a focus on understanding the toxin’s structure-function relationships and its interaction with nicotinic acetylcholine receptors . This research could potentially lead to the discovery of therapeutic lead compounds from snake venoms .
Preparation Methods
Synthetic Routes and Reaction Conditions
The synthesis of Naja mossambica mossambica alpha-neurotoxin I involves complex peptide synthesis techniques. Typically, solid-phase peptide synthesis (SPPS) is employed, where amino acids are sequentially added to a growing peptide chain anchored to a solid resin. The process includes multiple steps of deprotection and coupling reactions, often facilitated by reagents such as N,N’-diisopropylcarbodiimide (DIC) and hydroxybenzotriazole (HOBt). The final product is cleaved from the resin and purified using high-performance liquid chromatography (HPLC) .
Industrial Production Methods
Industrial production of this compound is not common due to the complexity and cost of synthesis. recombinant DNA technology can be used to produce the toxin in bacterial or yeast expression systems. This method involves inserting the gene encoding the neurotoxin into an expression vector, which is then introduced into the host organism. The host cells produce the neurotoxin, which is subsequently purified using chromatographic techniques .
Chemical Reactions Analysis
Types of Reactions
Naja mossambica mossambica alpha-neurotoxin I primarily undergoes peptide bond formation and cleavage reactions during its synthesis. It does not typically participate in oxidation, reduction, or substitution reactions under physiological conditions .
Common Reagents and Conditions
Common reagents used in the synthesis of this compound include DIC, HOBt, and trifluoroacetic acid (TFA) for deprotection steps. The synthesis is carried out under controlled conditions to ensure the correct folding and disulfide bond formation, which are crucial for the biological activity of the neurotoxin .
Major Products Formed
The major product formed from the synthesis of this compound is the fully folded and biologically active peptide. Any misfolded or truncated peptides are typically removed during the purification process .
Comparison with Similar Compounds
Similar Compounds
Alpha-bungarotoxin: Derived from the venom of the banded krait (Bungarus multicinctus), it also targets nicotinic acetylcholine receptors but has a different binding affinity and specificity.
Alpha-cobratoxin: Found in the venom of the Thai cobra (Naja kaouthia), it shares a similar mechanism of action but differs in its amino acid sequence and structural conformation.
Alpha-elapitoxin: Present in the venom of various elapid snakes, it exhibits similar neurotoxic effects but varies in its potency and receptor binding characteristics
Uniqueness
Naja mossambica mossambica alpha-neurotoxin I is unique due to its specific binding affinity to the nicotinic acetylcholine receptors and its structural conformation, which contributes to its potent neurotoxic effects. Its study has provided valuable insights into the structure-function relationships of neurotoxins and their interactions with ion channels .
Properties
IUPAC Name |
(2S)-1-[(2S)-1-[(2S,3S)-2-[[(2S)-2-amino-4-methylpentanoyl]amino]-3-methylpentanoyl]pyrrolidine-2-carbonyl]-N-[(2S)-1-[[(2S)-1-[[(2S)-1,6-diamino-1-oxohexan-2-yl]amino]-3-(1H-indol-3-yl)-1-oxopropan-2-yl]amino]-1-oxo-3-phenylpropan-2-yl]pyrrolidine-2-carboxamide | |
---|---|---|
Source | PubChem | |
URL | https://pubchem.ncbi.nlm.nih.gov | |
Description | Data deposited in or computed by PubChem | |
InChI |
InChI=1S/C48H70N10O7/c1-5-30(4)41(56-43(60)34(50)25-29(2)3)48(65)58-24-14-21-40(58)47(64)57-23-13-20-39(57)46(63)55-37(26-31-15-7-6-8-16-31)44(61)54-38(27-32-28-52-35-18-10-9-17-33(32)35)45(62)53-36(42(51)59)19-11-12-22-49/h6-10,15-18,28-30,34,36-41,52H,5,11-14,19-27,49-50H2,1-4H3,(H2,51,59)(H,53,62)(H,54,61)(H,55,63)(H,56,60)/t30-,34-,36-,37-,38-,39-,40-,41-/m0/s1 | |
Source | PubChem | |
URL | https://pubchem.ncbi.nlm.nih.gov | |
Description | Data deposited in or computed by PubChem | |
InChI Key |
IVORLIHJCUSAQL-USNVFINLSA-N | |
Source | PubChem | |
URL | https://pubchem.ncbi.nlm.nih.gov | |
Description | Data deposited in or computed by PubChem | |
Canonical SMILES |
CCC(C)C(C(=O)N1CCCC1C(=O)N2CCCC2C(=O)NC(CC3=CC=CC=C3)C(=O)NC(CC4=CNC5=CC=CC=C54)C(=O)NC(CCCCN)C(=O)N)NC(=O)C(CC(C)C)N | |
Source | PubChem | |
URL | https://pubchem.ncbi.nlm.nih.gov | |
Description | Data deposited in or computed by PubChem | |
Isomeric SMILES |
CC[C@H](C)[C@@H](C(=O)N1CCC[C@H]1C(=O)N2CCC[C@H]2C(=O)N[C@@H](CC3=CC=CC=C3)C(=O)N[C@@H](CC4=CNC5=CC=CC=C54)C(=O)N[C@@H](CCCCN)C(=O)N)NC(=O)[C@H](CC(C)C)N | |
Source | PubChem | |
URL | https://pubchem.ncbi.nlm.nih.gov | |
Description | Data deposited in or computed by PubChem | |
Molecular Formula |
C48H70N10O7 | |
Source | PubChem | |
URL | https://pubchem.ncbi.nlm.nih.gov | |
Description | Data deposited in or computed by PubChem | |
DSSTOX Substance ID |
DTXSID30151175 | |
Record name | Naja mossambica mossambica alpha-neurotoxin I | |
Source | EPA DSSTox | |
URL | https://comptox.epa.gov/dashboard/DTXSID30151175 | |
Description | DSSTox provides a high quality public chemistry resource for supporting improved predictive toxicology. | |
Molecular Weight |
899.1 g/mol | |
Source | PubChem | |
URL | https://pubchem.ncbi.nlm.nih.gov | |
Description | Data deposited in or computed by PubChem | |
CAS No. |
115722-23-1 | |
Record name | Naja mossambica mossambica alpha-neurotoxin I | |
Source | ChemIDplus | |
URL | https://pubchem.ncbi.nlm.nih.gov/substance/?source=chemidplus&sourceid=0115722231 | |
Description | ChemIDplus is a free, web search system that provides access to the structure and nomenclature authority files used for the identification of chemical substances cited in National Library of Medicine (NLM) databases, including the TOXNET system. | |
Record name | Naja mossambica mossambica alpha-neurotoxin I | |
Source | EPA DSSTox | |
URL | https://comptox.epa.gov/dashboard/DTXSID30151175 | |
Description | DSSTox provides a high quality public chemistry resource for supporting improved predictive toxicology. | |
Retrosynthesis Analysis
AI-Powered Synthesis Planning: Our tool employs the Template_relevance Pistachio, Template_relevance Bkms_metabolic, Template_relevance Pistachio_ringbreaker, Template_relevance Reaxys, Template_relevance Reaxys_biocatalysis model, leveraging a vast database of chemical reactions to predict feasible synthetic routes.
One-Step Synthesis Focus: Specifically designed for one-step synthesis, it provides concise and direct routes for your target compounds, streamlining the synthesis process.
Accurate Predictions: Utilizing the extensive PISTACHIO, BKMS_METABOLIC, PISTACHIO_RINGBREAKER, REAXYS, REAXYS_BIOCATALYSIS database, our tool offers high-accuracy predictions, reflecting the latest in chemical research and data.
Strategy Settings
Precursor scoring | Relevance Heuristic |
---|---|
Min. plausibility | 0.01 |
Model | Template_relevance |
Template Set | Pistachio/Bkms_metabolic/Pistachio_ringbreaker/Reaxys/Reaxys_biocatalysis |
Top-N result to add to graph | 6 |
Feasible Synthetic Routes
Disclaimer and Information on In-Vitro Research Products
Please be aware that all articles and product information presented on BenchChem are intended solely for informational purposes. The products available for purchase on BenchChem are specifically designed for in-vitro studies, which are conducted outside of living organisms. In-vitro studies, derived from the Latin term "in glass," involve experiments performed in controlled laboratory settings using cells or tissues. It is important to note that these products are not categorized as medicines or drugs, and they have not received approval from the FDA for the prevention, treatment, or cure of any medical condition, ailment, or disease. We must emphasize that any form of bodily introduction of these products into humans or animals is strictly prohibited by law. It is essential to adhere to these guidelines to ensure compliance with legal and ethical standards in research and experimentation.