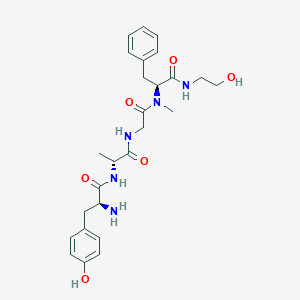
Damgo
Overview
Description
The compound “DAGO” is a synthetic opioid peptide known for its high specificity to the μ-opioid receptor. It was synthesized as a biologically stable analog of δ-opioid receptor-preferring endogenous opioids, such as leucine-enkephalin and methionine-enkephalin . The structure of DAGO is H-Tyr-D-Ala-Gly-N-MePhe-Gly-ol .
Scientific Research Applications
DAGO has a wide range of scientific research applications, including:
Chemistry: Used as a model compound for studying peptide synthesis and reactions.
Biology: Employed in research on opioid receptors and their role in pain modulation.
Medicine: Investigated for its potential in developing new analgesics with reduced tolerance and dependence.
Industry: Utilized in the production of peptide-based drugs and research reagents.
Mechanism of Action
DAMGO is a potent and selective μ-opioid receptor agonist . It suppresses activity in rNST neurons predominantly via a presynaptic mechanism . This compound, but not morphine, induces dimer formation in a process that correlates both temporally and in its agonist- and phosphorylation-dependence with β-arrestin2 binding to the receptors .
Safety and Hazards
Future Directions
The design of safer analgesics is a major research topic due to the notorious worldwide “opioid epidemic” . The identification of biased opioid ligands capable of eliciting selective functional responses has provided an alternative avenue to develop novel therapeutics without the side effects of current opioid medications . In the future, prolonged simulations of the MOR bound to fentanyl vs. NFEPP with different protonation of the drug and of the receptor could provide a glimpse into how protonation change shapes drug-receptor interactions at low pH of interest for pain treatment .
Biochemical Analysis
Biochemical Properties
“Damgo” plays a significant role in biochemical reactions, particularly in its interactions with μ-opioid receptors . It has a high affinity and selectivity for these receptors, making it a valuable tool in the study of opioid receptor function .
Cellular Effects
“this compound” exerts various effects on cells and cellular processes. It influences cell function by interacting with μ-opioid receptors, which are involved in a variety of cellular processes, including cell signaling pathways, gene expression, and cellular metabolism .
Molecular Mechanism
The molecular mechanism of “this compound” involves its binding to μ-opioid receptors . This binding interaction triggers a series of events at the molecular level, including changes in gene expression and enzyme activity .
Dosage Effects in Animal Models
The effects of “this compound” can vary with different dosages in animal models .
Metabolic Pathways
“this compound” is involved in various metabolic pathways, particularly those involving μ-opioid receptors . It interacts with enzymes and cofactors in these pathways, potentially affecting metabolic flux or metabolite levels .
Transport and Distribution
“this compound” is transported and distributed within cells and tissues via its interactions with μ-opioid receptors . These interactions can affect its localization or accumulation within cells .
Subcellular Localization
The subcellular localization of “this compound” is largely determined by its interactions with μ-opioid receptors . These interactions can direct “this compound” to specific compartments or organelles within the cell .
Preparation Methods
Synthetic Routes and Reaction Conditions: DAGO is synthesized through a series of peptide coupling reactions. The synthesis typically involves the stepwise addition of amino acids to form the peptide chain. The reaction conditions often include the use of coupling reagents such as dicyclohexylcarbodiimide (DCC) and N-hydroxysuccinimide (NHS) to facilitate the formation of peptide bonds .
Industrial Production Methods: Industrial production of DAGO involves large-scale peptide synthesis techniques. Solid-phase peptide synthesis (SPPS) is commonly used, where the peptide is assembled on a solid resin support. This method allows for efficient and automated synthesis of peptides with high purity and yield .
Chemical Reactions Analysis
Types of Reactions: DAGO undergoes various chemical reactions, including:
Oxidation: DAGO can be oxidized to form disulfide bonds between cysteine residues if present.
Reduction: Reduction reactions can break disulfide bonds, converting them back to thiol groups.
Substitution: DAGO can undergo substitution reactions where functional groups on the peptide are replaced with other groups.
Common Reagents and Conditions:
Oxidation: Hydrogen peroxide (H₂O₂) or iodine (I₂) can be used as oxidizing agents.
Reduction: Dithiothreitol (DTT) or tris(2-carboxyethyl)phosphine (TCEP) are common reducing agents.
Substitution: Various reagents such as alkyl halides or acyl chlorides can be used for substitution reactions.
Major Products Formed:
Oxidation: Formation of disulfide-linked peptides.
Reduction: Formation of free thiol-containing peptides.
Substitution: Formation of modified peptides with new functional groups.
Comparison with Similar Compounds
DAMGO: Another synthetic opioid peptide with high μ-opioid receptor specificity.
DPDPE: A synthetic peptide with high δ-opioid receptor specificity.
Morphiceptin: A tetrapeptide with high affinity for the μ-opioid receptor.
Comparison:
DAGO vs. This compound: Both have high μ-opioid receptor specificity, but DAGO is more stable and has a longer duration of action.
DAGO vs. DPDPE: DAGO targets the μ-opioid receptor, while DPDPE targets the δ-opioid receptor.
DAGO vs. Morphiceptin: DAGO has a more complex structure and higher receptor specificity compared to Morphiceptin.
DAGO’s uniqueness lies in its high specificity and stability, making it a valuable compound for research and potential therapeutic applications .
Properties
IUPAC Name |
(2S)-2-amino-N-[(2R)-1-[[2-[[(2S)-1-(2-hydroxyethylamino)-1-oxo-3-phenylpropan-2-yl]-methylamino]-2-oxoethyl]amino]-1-oxopropan-2-yl]-3-(4-hydroxyphenyl)propanamide | |
---|---|---|
Source | PubChem | |
URL | https://pubchem.ncbi.nlm.nih.gov | |
Description | Data deposited in or computed by PubChem | |
InChI |
InChI=1S/C26H35N5O6/c1-17(30-25(36)21(27)14-19-8-10-20(33)11-9-19)24(35)29-16-23(34)31(2)22(26(37)28-12-13-32)15-18-6-4-3-5-7-18/h3-11,17,21-22,32-33H,12-16,27H2,1-2H3,(H,28,37)(H,29,35)(H,30,36)/t17-,21+,22+/m1/s1 | |
Source | PubChem | |
URL | https://pubchem.ncbi.nlm.nih.gov | |
Description | Data deposited in or computed by PubChem | |
InChI Key |
HPZJMUBDEAMBFI-WTNAPCKOSA-N | |
Source | PubChem | |
URL | https://pubchem.ncbi.nlm.nih.gov | |
Description | Data deposited in or computed by PubChem | |
Canonical SMILES |
CC(C(=O)NCC(=O)N(C)C(CC1=CC=CC=C1)C(=O)NCCO)NC(=O)C(CC2=CC=C(C=C2)O)N | |
Source | PubChem | |
URL | https://pubchem.ncbi.nlm.nih.gov | |
Description | Data deposited in or computed by PubChem | |
Isomeric SMILES |
C[C@H](C(=O)NCC(=O)N(C)[C@@H](CC1=CC=CC=C1)C(=O)NCCO)NC(=O)[C@H](CC2=CC=C(C=C2)O)N | |
Source | PubChem | |
URL | https://pubchem.ncbi.nlm.nih.gov | |
Description | Data deposited in or computed by PubChem | |
Molecular Formula |
C26H35N5O6 | |
Source | PubChem | |
URL | https://pubchem.ncbi.nlm.nih.gov | |
Description | Data deposited in or computed by PubChem | |
DSSTOX Substance ID |
DTXSID30228775 | |
Record name | L-Tyrosyl-D-alanylglycyl-N-(2-hydroxyethyl)-Nalpha-methyl-L-phenylalaninamide | |
Source | EPA DSSTox | |
URL | https://comptox.epa.gov/dashboard/DTXSID30228775 | |
Description | DSSTox provides a high quality public chemistry resource for supporting improved predictive toxicology. | |
Molecular Weight |
513.6 g/mol | |
Source | PubChem | |
URL | https://pubchem.ncbi.nlm.nih.gov | |
Description | Data deposited in or computed by PubChem | |
CAS No. |
78123-71-4 | |
Record name | Enkephalin, ala(2)-mephe(4)-gly(5)- | |
Source | ChemIDplus | |
URL | https://pubchem.ncbi.nlm.nih.gov/substance/?source=chemidplus&sourceid=0078123714 | |
Description | ChemIDplus is a free, web search system that provides access to the structure and nomenclature authority files used for the identification of chemical substances cited in National Library of Medicine (NLM) databases, including the TOXNET system. | |
Record name | L-Tyrosyl-D-alanylglycyl-N-(2-hydroxyethyl)-Nalpha-methyl-L-phenylalaninamide | |
Source | EPA DSSTox | |
URL | https://comptox.epa.gov/dashboard/DTXSID30228775 | |
Description | DSSTox provides a high quality public chemistry resource for supporting improved predictive toxicology. | |
Record name | DAMGO | |
Source | FDA Global Substance Registration System (GSRS) | |
URL | https://gsrs.ncats.nih.gov/ginas/app/beta/substances/2YM5VT3MNR | |
Description | The FDA Global Substance Registration System (GSRS) enables the efficient and accurate exchange of information on what substances are in regulated products. Instead of relying on names, which vary across regulatory domains, countries, and regions, the GSRS knowledge base makes it possible for substances to be defined by standardized, scientific descriptions. | |
Explanation | Unless otherwise noted, the contents of the FDA website (www.fda.gov), both text and graphics, are not copyrighted. They are in the public domain and may be republished, reprinted and otherwise used freely by anyone without the need to obtain permission from FDA. Credit to the U.S. Food and Drug Administration as the source is appreciated but not required. | |
Retrosynthesis Analysis
AI-Powered Synthesis Planning: Our tool employs the Template_relevance Pistachio, Template_relevance Bkms_metabolic, Template_relevance Pistachio_ringbreaker, Template_relevance Reaxys, Template_relevance Reaxys_biocatalysis model, leveraging a vast database of chemical reactions to predict feasible synthetic routes.
One-Step Synthesis Focus: Specifically designed for one-step synthesis, it provides concise and direct routes for your target compounds, streamlining the synthesis process.
Accurate Predictions: Utilizing the extensive PISTACHIO, BKMS_METABOLIC, PISTACHIO_RINGBREAKER, REAXYS, REAXYS_BIOCATALYSIS database, our tool offers high-accuracy predictions, reflecting the latest in chemical research and data.
Strategy Settings
Precursor scoring | Relevance Heuristic |
---|---|
Min. plausibility | 0.01 |
Model | Template_relevance |
Template Set | Pistachio/Bkms_metabolic/Pistachio_ringbreaker/Reaxys/Reaxys_biocatalysis |
Top-N result to add to graph | 6 |
Feasible Synthetic Routes
Disclaimer and Information on In-Vitro Research Products
Please be aware that all articles and product information presented on BenchChem are intended solely for informational purposes. The products available for purchase on BenchChem are specifically designed for in-vitro studies, which are conducted outside of living organisms. In-vitro studies, derived from the Latin term "in glass," involve experiments performed in controlled laboratory settings using cells or tissues. It is important to note that these products are not categorized as medicines or drugs, and they have not received approval from the FDA for the prevention, treatment, or cure of any medical condition, ailment, or disease. We must emphasize that any form of bodily introduction of these products into humans or animals is strictly prohibited by law. It is essential to adhere to these guidelines to ensure compliance with legal and ethical standards in research and experimentation.