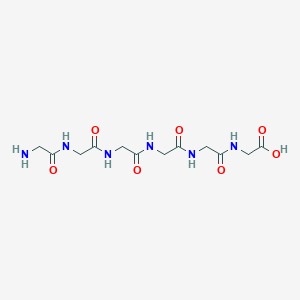
Hexaglycine
Overview
Description
Glycine is the simplest amino acid, and its repetitive sequence in Gly6 results in a molecule with the formula C12H20N6O7 and a molecular weight of 360.32 g/mol . Gly6 is primarily used in research settings and is known for its role in various biochemical and biophysical studies.
Preparation Methods
Synthetic Routes and Reaction Conditions: Gly6 can be synthesized using standard solid-phase peptide synthesis (SPPS) techniques. The process involves the sequential addition of glycine residues to a growing peptide chain anchored to a solid resin. The synthesis typically employs Fmoc (9-fluorenylmethyloxycarbonyl) chemistry for temporary protection of the amino group during the coupling reactions. The general steps include:
Deprotection: Removal of the Fmoc group using a base such as piperidine.
Coupling: Addition of the next glycine residue using a coupling reagent like HBTU (O-benzotriazole-N,N,N’,N’-tetramethyluronium hexafluorophosphate) in the presence of a base like DIPEA (N,N-diisopropylethylamine).
Cleavage: Final cleavage of the peptide from the resin using a mixture of trifluoroacetic acid (TFA), water, and scavengers like triisopropylsilane (TIS).
Industrial Production Methods: While Gly6 is primarily synthesized for research purposes, industrial-scale production would follow similar SPPS techniques with optimization for larger batch sizes. Automation and high-throughput peptide synthesizers can be employed to increase efficiency and yield.
Chemical Reactions Analysis
Types of Reactions: Gly6 can undergo various chemical reactions, including:
Hydrolysis: Breaking down into individual glycine residues in the presence of strong acids or bases.
Oxidation: Glycine residues can be oxidized to form glyoxylate and ammonia under specific conditions.
Substitution: The amino groups in Gly6 can participate in substitution reactions with electrophiles.
Common Reagents and Conditions:
Hydrolysis: Typically performed using hydrochloric acid or sodium hydroxide at elevated temperatures.
Oxidation: Can be achieved using oxidizing agents like hydrogen peroxide or potassium permanganate.
Substitution: Electrophilic reagents such as acyl chlorides or isocyanates can be used under mild conditions.
Major Products:
Hydrolysis: Produces free glycine residues.
Oxidation: Results in glyoxylate and ammonia.
Substitution: Forms various substituted glycine derivatives depending on the electrophile used.
Scientific Research Applications
Gly6 has a wide range of applications in scientific research:
Biochemistry: Used as a model peptide to study protein folding, stability, and interactions.
Biophysics: Employed in experiments to understand peptide dynamics and conformational changes.
Medicine: Investigated for its potential role in drug delivery systems and as a building block for therapeutic peptides.
Industry: Utilized in the development of peptide-based materials and as a substrate in enzymatic studies.
Mechanism of Action
The mechanism of action of Gly6 is primarily related to its structural properties as a peptide. It can interact with various biological molecules through hydrogen bonding, electrostatic interactions, and hydrophobic effects. In enzymatic studies, Gly6 serves as a substrate for proteases, which cleave the peptide bonds between glycine residues. The cleavage products can then participate in further biochemical pathways .
Comparison with Similar Compounds
Gly2 (diglycine): Composed of two glycine residues.
Gly3 (triglycine): Composed of three glycine residues.
Gly4 (tetraglycine): Composed of four glycine residues.
Gly5 (pentaglycine): Composed of five glycine residues.
Comparison:
Length and Complexity: Gly6 is longer and more complex than its shorter counterparts, which can influence its solubility, stability, and interactions with other molecules.
Biological Activity: The increased length of Gly6 allows for more extensive interactions with biological targets, potentially enhancing its utility in research and therapeutic applications.
Unique Properties: Gly6’s repetitive glycine sequence provides a unique model for studying peptide behavior and interactions, making it distinct from shorter glycine peptides.
Properties
IUPAC Name |
2-[[2-[[2-[[2-[[2-[(2-aminoacetyl)amino]acetyl]amino]acetyl]amino]acetyl]amino]acetyl]amino]acetic acid | |
---|---|---|
Source | PubChem | |
URL | https://pubchem.ncbi.nlm.nih.gov | |
Description | Data deposited in or computed by PubChem | |
InChI |
InChI=1S/C12H20N6O7/c13-1-7(19)14-2-8(20)15-3-9(21)16-4-10(22)17-5-11(23)18-6-12(24)25/h1-6,13H2,(H,14,19)(H,15,20)(H,16,21)(H,17,22)(H,18,23)(H,24,25) | |
Source | PubChem | |
URL | https://pubchem.ncbi.nlm.nih.gov | |
Description | Data deposited in or computed by PubChem | |
InChI Key |
XJFPXLWGZWAWRQ-UHFFFAOYSA-N | |
Source | PubChem | |
URL | https://pubchem.ncbi.nlm.nih.gov | |
Description | Data deposited in or computed by PubChem | |
Canonical SMILES |
C(C(=O)NCC(=O)NCC(=O)NCC(=O)NCC(=O)NCC(=O)O)N | |
Source | PubChem | |
URL | https://pubchem.ncbi.nlm.nih.gov | |
Description | Data deposited in or computed by PubChem | |
Molecular Formula |
C12H20N6O7 | |
Source | PubChem | |
URL | https://pubchem.ncbi.nlm.nih.gov | |
Description | Data deposited in or computed by PubChem | |
DSSTOX Substance ID |
DTXSID90192125 | |
Record name | Hexaglycine | |
Source | EPA DSSTox | |
URL | https://comptox.epa.gov/dashboard/DTXSID90192125 | |
Description | DSSTox provides a high quality public chemistry resource for supporting improved predictive toxicology. | |
Molecular Weight |
360.32 g/mol | |
Source | PubChem | |
URL | https://pubchem.ncbi.nlm.nih.gov | |
Description | Data deposited in or computed by PubChem | |
CAS No. |
3887-13-6 | |
Record name | Hexaglycine | |
Source | ChemIDplus | |
URL | https://pubchem.ncbi.nlm.nih.gov/substance/?source=chemidplus&sourceid=0003887136 | |
Description | ChemIDplus is a free, web search system that provides access to the structure and nomenclature authority files used for the identification of chemical substances cited in National Library of Medicine (NLM) databases, including the TOXNET system. | |
Record name | 3887-13-6 | |
Source | DTP/NCI | |
URL | https://dtp.cancer.gov/dtpstandard/servlet/dwindex?searchtype=NSC&outputformat=html&searchlist=402061 | |
Description | The NCI Development Therapeutics Program (DTP) provides services and resources to the academic and private-sector research communities worldwide to facilitate the discovery and development of new cancer therapeutic agents. | |
Explanation | Unless otherwise indicated, all text within NCI products is free of copyright and may be reused without our permission. Credit the National Cancer Institute as the source. | |
Record name | Hexaglycine | |
Source | EPA DSSTox | |
URL | https://comptox.epa.gov/dashboard/DTXSID90192125 | |
Description | DSSTox provides a high quality public chemistry resource for supporting improved predictive toxicology. | |
Record name | HEXAGLYCINE | |
Source | FDA Global Substance Registration System (GSRS) | |
URL | https://gsrs.ncats.nih.gov/ginas/app/beta/substances/53RE43SN65 | |
Description | The FDA Global Substance Registration System (GSRS) enables the efficient and accurate exchange of information on what substances are in regulated products. Instead of relying on names, which vary across regulatory domains, countries, and regions, the GSRS knowledge base makes it possible for substances to be defined by standardized, scientific descriptions. | |
Explanation | Unless otherwise noted, the contents of the FDA website (www.fda.gov), both text and graphics, are not copyrighted. They are in the public domain and may be republished, reprinted and otherwise used freely by anyone without the need to obtain permission from FDA. Credit to the U.S. Food and Drug Administration as the source is appreciated but not required. | |
Retrosynthesis Analysis
AI-Powered Synthesis Planning: Our tool employs the Template_relevance Pistachio, Template_relevance Bkms_metabolic, Template_relevance Pistachio_ringbreaker, Template_relevance Reaxys, Template_relevance Reaxys_biocatalysis model, leveraging a vast database of chemical reactions to predict feasible synthetic routes.
One-Step Synthesis Focus: Specifically designed for one-step synthesis, it provides concise and direct routes for your target compounds, streamlining the synthesis process.
Accurate Predictions: Utilizing the extensive PISTACHIO, BKMS_METABOLIC, PISTACHIO_RINGBREAKER, REAXYS, REAXYS_BIOCATALYSIS database, our tool offers high-accuracy predictions, reflecting the latest in chemical research and data.
Strategy Settings
Precursor scoring | Relevance Heuristic |
---|---|
Min. plausibility | 0.01 |
Model | Template_relevance |
Template Set | Pistachio/Bkms_metabolic/Pistachio_ringbreaker/Reaxys/Reaxys_biocatalysis |
Top-N result to add to graph | 6 |
Feasible Synthetic Routes
Q1: What is the molecular formula and weight of Hexaglycine?
A1: this compound, with six glycine residues (C2H5NO2) linked together, possesses a molecular formula of C12H20N6O7 and a molecular weight of 360.31 g/mol.
Q2: Is there spectroscopic data available for this compound?
A2: Yes, studies utilizing techniques like circular dichroism (CD) spectroscopy have provided insights into the conformational behavior of this compound and its analogs. For instance, while exhibiting a random coil conformation in water, an analog of ACTH-(1-24)-tetracosapeptide containing this compound displayed alpha-helical characteristics in trifluoroethanol, highlighting the impact of solvent environment on its structure [].
Q3: How does the flexibility of this compound influence its end-to-end distance distribution?
A3: Research employing frequency-domain fluorescence spectroscopy has revealed that the flexibility of the peptide chain significantly impacts the end-to-end distance distribution in this compound. Specifically, the flexible nature of glycine results in a higher degree of conformational heterogeneity compared to more rigid peptides like Hexaproline. This difference in flexibility translates to a wider distribution of distances between the donor and acceptor molecules attached at the ends of the this compound chain [].
Q4: How does the presence of this compound in a fusion protein affect the protein's half-life?
A4: Studies have shown that incorporating this compound as a linker in a reiterated form of rabbit serum albumin (RSA) did not significantly alter the protein's half-life compared to a single unit of RSA []. This suggests that while albumin itself can extend the half-life of smaller proteins, simply reiterating it with a this compound linker might not confer additional benefits in terms of half-life extension.
Q5: Is the this compound peptide bond stable under strong alkaline conditions during peptide synthesis?
A5: Yes, a key advantage of using the bulky ferrocenylmethyl (Fem) group to protect glycine residues during peptide synthesis is the remarkable stability of the Gly-Gly bond in the presence of strong alkaline conditions, typically encountered during methyl ester hydrolysis steps []. This stability ensures high yields throughout the synthesis process.
Q6: Can this compound be used as a substrate to study the activity of lysostaphin-like bacteriolytic agents?
A6: Yes, this compound serves as a valuable tool for investigating the mode of action of bacteriolytic agents like zoocin A, produced by Streptococcus zooepidemicus. By observing zoocin A's ability to cleave this compound in a cell-free system, researchers have gathered evidence supporting its lysostaphin-like activity, suggesting that zoocin A targets and cleaves peptidoglycan cross-links in bacterial cell walls [].
Q7: How does the length of glycine oligopeptides affect their hydrolysis by intestinal enzymes?
A7: Studies on guinea pig intestinal mucosa have shown a correlation between the length of glycine oligopeptides and their susceptibility to enzymatic hydrolysis. While the mucosa exhibited hydrolytic activity for oligopeptides up to this compound, it did not show any activity against larger homopolypeptides []. This suggests a size limitation for the peptidase enzymes involved in the hydrolysis of glycine chains.
Q8: What is the role of this compound in studying the transport of glycine peptides in the human jejunum?
A8: Research using this compound has been crucial in determining the limits of intact absorption of glycine oligopeptides in the human jejunum. Results indicate that while di- and triglycine are readily absorbed, the uptake of glycine from this compound is comparable to that from free glycine. This suggests that longer glycine peptides are primarily hydrolyzed before absorption, highlighting the importance of peptide length for efficient nutrient uptake in the gut [].
Q9: How is this compound used in computational chemistry for studying peptide aggregation?
A9: this compound serves as a model peptide for investigating the aggregation behavior of polypeptide chains using density functional theory (DFT) calculations. By simulating the interactions of this compound strands in various conformations, researchers can gain insights into the energetic contributions of hydrogen bonding and structural distortions to the stability of different aggregated forms, such as pleated and rippled beta-sheets, collagen-like structures, and polyglycine helices [].
Q10: Can genetic algorithms be used to determine the low-energy conformations of this compound?
A10: Yes, genetic algorithms (GA) have been successfully employed to explore the conformational space of cyclic this compound and identify its low-energy conformations []. This approach involves utilizing GA parameters such as population size, number of generations, and interaction rates to effectively search for energetically favorable structures within a computationally feasible timeframe.
Disclaimer and Information on In-Vitro Research Products
Please be aware that all articles and product information presented on BenchChem are intended solely for informational purposes. The products available for purchase on BenchChem are specifically designed for in-vitro studies, which are conducted outside of living organisms. In-vitro studies, derived from the Latin term "in glass," involve experiments performed in controlled laboratory settings using cells or tissues. It is important to note that these products are not categorized as medicines or drugs, and they have not received approval from the FDA for the prevention, treatment, or cure of any medical condition, ailment, or disease. We must emphasize that any form of bodily introduction of these products into humans or animals is strictly prohibited by law. It is essential to adhere to these guidelines to ensure compliance with legal and ethical standards in research and experimentation.