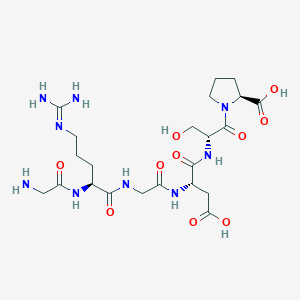
Grgdsp
Overview
Description
Gly-Arg-Gly-Asp-Ser-Pro (GRGDSP) is a bioactive peptide derived from fibronectin. It is known for its ability to promote cell adhesion by interacting with integrin receptors on the cell surface . This peptide sequence is widely used in biomedical research and applications due to its role in enhancing cell attachment and proliferation.
Preparation Methods
Synthetic Routes and Reaction Conditions
GRGDSP can be synthesized using solid-phase peptide synthesis (SPPS), a common method for producing peptides. The synthesis involves sequentially adding protected amino acids to a growing peptide chain anchored to a solid resin. The protecting groups are removed, and the peptide is cleaved from the resin, followed by purification using high-performance liquid chromatography (HPLC) .
Industrial Production Methods
In industrial settings, the synthesis of this compound may involve automated peptide synthesizers to increase efficiency and yield. The process includes the use of coupling reagents, such as N,N’-diisopropylcarbodiimide (DIC) and hydroxybenzotriazole (HOBt), to facilitate the formation of peptide bonds .
Chemical Reactions Analysis
Types of Reactions
GRGDSP primarily undergoes reactions typical of peptides, including:
Oxidation: The peptide can undergo oxidation, particularly at the methionine residue if present.
Reduction: Disulfide bonds, if any, can be reduced using reducing agents like dithiothreitol (DTT).
Substitution: Amino acid residues in the peptide can be substituted to create analogs with different properties.
Common Reagents and Conditions
Oxidation: Hydrogen peroxide or other oxidizing agents.
Reduction: Dithiothreitol (DTT) or tris(2-carboxyethyl)phosphine (TCEP).
Substitution: Various amino acid derivatives and coupling reagents.
Major Products Formed
The major products formed from these reactions include oxidized or reduced forms of the peptide and substituted analogs with modified biological activities .
Scientific Research Applications
GRGDSP has a wide range of applications in scientific research:
Chemistry: Used as a model peptide for studying peptide synthesis and modification techniques.
Biology: Facilitates cell adhesion studies by interacting with integrin receptors on cell surfaces.
Medicine: Enhances the biocompatibility of medical implants by promoting endothelialization.
Mechanism of Action
GRGDSP exerts its effects by binding to integrin receptors on the cell surface. This interaction triggers intracellular signaling pathways, including the activation of mitogen-activated protein kinase (MAPK) and increases in intracellular calcium levels . These signaling events promote cell adhesion, proliferation, and survival .
Comparison with Similar Compounds
Similar Compounds
Gly-Arg-Gly-Asp-Ser (GRGDS): Another peptide with similar cell adhesion properties.
Gly-Arg-Gly-Asp-Ser-Pro-Lys (GRGDSPK): An extended version of GRGDSP with additional lysine for enhanced solubility.
Uniqueness
This compound is unique due to its specific sequence that optimally promotes cell adhesion and proliferation. Its ability to be easily synthesized and modified makes it a versatile tool in various research and industrial applications .
Biological Activity
The GRGDSP peptide, derived from the fibronectin sequence, is a crucial integrin-binding motif that plays a significant role in cell adhesion, migration, and proliferation. This article explores the biological activity of this compound, emphasizing its applications in biomedical research, particularly in tissue engineering and drug delivery systems.
Overview of this compound
This compound (Gly-Arg-Gly-Asp-Ser-Pro) is a pentapeptide that specifically interacts with various integrin receptors on cell surfaces. The RGD sequence has been identified as a minimal binding motif for integrins, which are proteins that facilitate cell-extracellular matrix (ECM) interactions. The biological activity of this compound is primarily mediated through its binding to integrins such as αvβ3, α5β1, and αIIbβ3, which are involved in processes like angiogenesis, wound healing, and tumor progression .
-
Cell Adhesion and Spreading :
- Studies have shown that immobilized this compound enhances endothelial cell (EC) adhesion and spreading on surfaces. The density of this compound significantly influences these processes; for instance, EC adhesion increased with surface density up to a certain point before plateauing .
- A notable finding indicates that a this compound density of 0.2 pmol/cm² can activate extracellular signal-regulated kinase (ERK), highlighting its role in cellular signaling pathways critical for proliferation and migration .
-
Cell Migration :
- The migration of ECs on this compound-modified surfaces exhibits complex behavior. At lower densities, EC migration is facilitated; however, as the density increases beyond 0.8 pmol/cm², migration speed decreases due to larger focal adhesions formed under shear stress conditions . This suggests a catch-bond characteristic where increased ligand density alters integrin binding dynamics.
-
Induction of Smooth Muscle Cell Differentiation :
- This compound has been incorporated into poly(ethylene glycol) diacrylate (PEGDA) hydrogels to promote the re-differentiation of vascular smooth muscle cells (SMCs). Research indicates that RGD-gels support significant upregulation of contractile markers like smooth muscle α-actin and calponin, demonstrating the peptide's efficacy in guiding cell phenotype changes in tissue engineering applications .
1. Cardiovascular Implants
This compound is utilized to modify the surfaces of cardiovascular implants to enhance endothelialization. By promoting cell adhesion and proliferation, this compound-coated surfaces can reduce thrombogenesis risks associated with vascular grafts .
2. Cancer Targeting
RGD peptides are being explored for targeted cancer therapies due to their ability to bind specifically to integrins overexpressed in tumor vasculature. This targeting improves drug delivery efficiency and reduces side effects associated with conventional therapies .
3. 3D Bioprinting
Incorporating this compound into bioinks has enabled enhanced cell attachment and viability in 3D bioprinted constructs, paving the way for more effective tissue engineering strategies .
Data Tables
Case Studies
- Case Study 1: Surface Modification for Vascular Grafts
- Case Study 2: RGD Peptides in Tumor Targeting
Properties
IUPAC Name |
(2S)-1-[(2S)-2-[[(2S)-2-[[2-[[(2S)-2-[(2-aminoacetyl)amino]-5-(diaminomethylideneamino)pentanoyl]amino]acetyl]amino]-3-carboxypropanoyl]amino]-3-hydroxypropanoyl]pyrrolidine-2-carboxylic acid | |
---|---|---|
Source | PubChem | |
URL | https://pubchem.ncbi.nlm.nih.gov | |
Description | Data deposited in or computed by PubChem | |
InChI |
InChI=1S/C22H37N9O10/c23-8-15(33)28-11(3-1-5-26-22(24)25)18(37)27-9-16(34)29-12(7-17(35)36)19(38)30-13(10-32)20(39)31-6-2-4-14(31)21(40)41/h11-14,32H,1-10,23H2,(H,27,37)(H,28,33)(H,29,34)(H,30,38)(H,35,36)(H,40,41)(H4,24,25,26)/t11-,12-,13-,14-/m0/s1 | |
Source | PubChem | |
URL | https://pubchem.ncbi.nlm.nih.gov | |
Description | Data deposited in or computed by PubChem | |
InChI Key |
NTEDOEBWPRVVSG-XUXIUFHCSA-N | |
Source | PubChem | |
URL | https://pubchem.ncbi.nlm.nih.gov | |
Description | Data deposited in or computed by PubChem | |
Canonical SMILES |
C1CC(N(C1)C(=O)C(CO)NC(=O)C(CC(=O)O)NC(=O)CNC(=O)C(CCCN=C(N)N)NC(=O)CN)C(=O)O | |
Source | PubChem | |
URL | https://pubchem.ncbi.nlm.nih.gov | |
Description | Data deposited in or computed by PubChem | |
Isomeric SMILES |
C1C[C@H](N(C1)C(=O)[C@H](CO)NC(=O)[C@H](CC(=O)O)NC(=O)CNC(=O)[C@H](CCCN=C(N)N)NC(=O)CN)C(=O)O | |
Source | PubChem | |
URL | https://pubchem.ncbi.nlm.nih.gov | |
Description | Data deposited in or computed by PubChem | |
Molecular Formula |
C22H37N9O10 | |
Source | PubChem | |
URL | https://pubchem.ncbi.nlm.nih.gov | |
Description | Data deposited in or computed by PubChem | |
Molecular Weight |
587.6 g/mol | |
Source | PubChem | |
URL | https://pubchem.ncbi.nlm.nih.gov | |
Description | Data deposited in or computed by PubChem | |
Retrosynthesis Analysis
AI-Powered Synthesis Planning: Our tool employs the Template_relevance Pistachio, Template_relevance Bkms_metabolic, Template_relevance Pistachio_ringbreaker, Template_relevance Reaxys, Template_relevance Reaxys_biocatalysis model, leveraging a vast database of chemical reactions to predict feasible synthetic routes.
One-Step Synthesis Focus: Specifically designed for one-step synthesis, it provides concise and direct routes for your target compounds, streamlining the synthesis process.
Accurate Predictions: Utilizing the extensive PISTACHIO, BKMS_METABOLIC, PISTACHIO_RINGBREAKER, REAXYS, REAXYS_BIOCATALYSIS database, our tool offers high-accuracy predictions, reflecting the latest in chemical research and data.
Strategy Settings
Precursor scoring | Relevance Heuristic |
---|---|
Min. plausibility | 0.01 |
Model | Template_relevance |
Template Set | Pistachio/Bkms_metabolic/Pistachio_ringbreaker/Reaxys/Reaxys_biocatalysis |
Top-N result to add to graph | 6 |
Feasible Synthetic Routes
Disclaimer and Information on In-Vitro Research Products
Please be aware that all articles and product information presented on BenchChem are intended solely for informational purposes. The products available for purchase on BenchChem are specifically designed for in-vitro studies, which are conducted outside of living organisms. In-vitro studies, derived from the Latin term "in glass," involve experiments performed in controlled laboratory settings using cells or tissues. It is important to note that these products are not categorized as medicines or drugs, and they have not received approval from the FDA for the prevention, treatment, or cure of any medical condition, ailment, or disease. We must emphasize that any form of bodily introduction of these products into humans or animals is strictly prohibited by law. It is essential to adhere to these guidelines to ensure compliance with legal and ethical standards in research and experimentation.