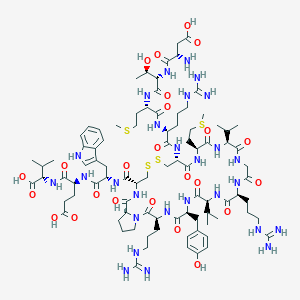
H-Asp-Thr-Met-Arg-Cys(1)-Met-Val-Gly-Arg-Val-Tyr-Arg-Pro-Cys(1)-Trp-Glu-Val-OH
Overview
Description
Melanin-concentrating hormone (MCH) is a cyclic neuropeptide originally isolated from the pituitary glands of chum salmon. It is composed of 19 amino acids and plays a significant role in regulating various physiological functions, including food intake, energy balance, sleep-wake cycles, and cardiovascular functions . In fish, MCH is primarily involved in melanin aggregation within melanophores, contributing to adaptive color changes .
Preparation Methods
Synthetic Routes and Reaction Conditions
The synthesis of MCH involves solid-phase peptide synthesis (SPPS), a method commonly used for the production of peptides. The process begins with the attachment of the C-terminal amino acid to a solid resin, followed by the sequential addition of protected amino acids. Each amino acid is coupled using reagents such as N,N’-diisopropylcarbodiimide (DIC) and hydroxybenzotriazole (HOBt). After the assembly of the peptide chain, the peptide is cleaved from the resin and deprotected using trifluoroacetic acid (TFA) to yield the final product .
Industrial Production Methods
Industrial production of MCH typically involves large-scale SPPS, followed by purification using high-performance liquid chromatography (HPLC). The purified peptide is then lyophilized to obtain a stable powder form. Quality control measures, including mass spectrometry and amino acid analysis, are employed to ensure the purity and identity of the peptide .
Chemical Reactions Analysis
Types of Reactions
MCH undergoes various chemical reactions, including:
Oxidation: The disulfide bond between cysteine residues can be oxidized, affecting the peptide’s conformation and activity.
Reduction: Reduction of the disulfide bond can lead to the linearization of the cyclic peptide.
Substitution: Amino acid residues within the peptide can be substituted to study structure-activity relationships.
Common Reagents and Conditions
Oxidation: Hydrogen peroxide (H2O2) or performic acid can be used for oxidation reactions.
Reduction: Dithiothreitol (DTT) or β-mercaptoethanol are commonly used reducing agents.
Substitution: Site-directed mutagenesis or chemical synthesis methods are employed to introduce specific amino acid substitutions.
Major Products Formed
Oxidation: Oxidized MCH with altered disulfide bonds.
Reduction: Linearized MCH without disulfide bonds.
Substitution: MCH analogs with modified amino acid sequences.
Scientific Research Applications
MCH has a wide range of scientific research applications:
Chemistry: Used as a model peptide for studying peptide synthesis, folding, and structure-activity relationships.
Industry: Utilized in the development of peptide-based drugs and as a research tool in neuroendocrinology.
Mechanism of Action
MCH exerts its effects by binding to specific G protein-coupled receptors, namely MCHR1 and MCHR2. The binding of MCH to these receptors triggers conformational changes, initiating intracellular signaling cascades via heterotrimeric G proteins. MCHR1 predominantly couples with inhibitory G proteins (Gi/o), while MCHR2 couples with Gq/11 . These signaling pathways regulate various physiological processes, including appetite, energy balance, and mood modulation .
Comparison with Similar Compounds
Similar Compounds
α-Melanocyte-stimulating hormone (α-MSH): Involved in skin pigmentation and energy homeostasis.
Neuropeptide Y (NPY): Regulates feeding behavior and energy balance.
Uniqueness of MCH
MCH is unique in its dual role in both melanin aggregation in fish and the regulation of energy homeostasis and feeding behavior in mammals. Unlike α-MSH, which promotes melanin dispersion, MCH induces melanin aggregation, highlighting its distinct physiological functions .
Biological Activity
The compound H-Asp-Thr-Met-Arg-Cys(1)-Met-Val-Gly-Arg-Val-Tyr-Arg-Pro-Cys(1)-Trp-Glu-Val-OH is a synthetic peptide with a complex sequence of amino acids, which suggests potential biological activities. This article explores its biological activity, including mechanisms of action, pharmacological effects, and relevant case studies.
Chemical Structure and Properties
The chemical structure of this compound consists of 15 amino acids, with specific residues that may confer unique biological properties. The presence of cysteine residues (Cys) indicates potential for disulfide bond formation, which can stabilize the peptide's conformation and enhance its biological activity. The molecular formula is with a molecular weight of approximately 2,000 Da .
Antinociceptive Effects
Peptides similar to this compound have been studied for their potential antinociceptive effects. For example, modifications in peptide sequences have shown to enhance their binding affinity to opioid receptors, suggesting that this compound may also interact with such receptors to modulate pain perception .
Neuroprotective Effects
Some peptides are known to exhibit neuroprotective effects by reducing neuroinflammation and promoting neuronal survival. The presence of specific amino acids like Trp and Arg in the sequence may enhance neuroprotective properties through modulation of signaling pathways involved in neuroinflammation and apoptosis .
Pharmacological Evaluation
A study evaluating various bioactive peptides demonstrated that modifications in amino acid sequences can significantly influence their biological activity. Peptides similar to this compound were tested for their efficacy in reducing oxidative stress markers in cellular models. Results indicated that certain structural motifs were crucial for enhancing antioxidant capacity .
In Vivo Studies
In vivo studies have shown that peptides with sequences containing Arg and Cys exhibit enhanced bioavailability and therapeutic effects. For instance, a peptide derived from marine sources demonstrated significant antihypertensive effects in animal models, suggesting that similar peptides could be explored for cardiovascular benefits .
Comparative Analysis
The following table summarizes the biological activities of various peptides with similar structures:
Peptide Sequence | Antioxidant Activity | Antinociceptive Activity | Neuroprotective Effects |
---|---|---|---|
H-Asp-Thr-Met-Arg-Cys(1)-... | Potential | Potential | Potential |
Val-Val-Tyr-Pro-Trp-Thr-Dap-NH2 | High | Moderate | High |
Gly-Ala-Glu-Arg-Pro | Moderate | Low | Moderate |
Properties
IUPAC Name |
(4S)-4-[[(2S)-2-[[(3S,6S,9S,12S,18S,21S,24R,29R,32S)-24-[[(2S)-2-[[(2S)-2-[[(2S,3R)-2-[[(2S)-2-amino-3-carboxypropanoyl]amino]-3-hydroxybutanoyl]amino]-4-methylsulfanylbutanoyl]amino]-5-carbamimidamidopentanoyl]amino]-3,12-bis(3-carbamimidamidopropyl)-6-[(4-hydroxyphenyl)methyl]-21-(2-methylsulfanylethyl)-2,5,8,11,14,17,20,23,31-nonaoxo-9,18-di(propan-2-yl)-26,27-dithia-1,4,7,10,13,16,19,22,30-nonazabicyclo[30.3.0]pentatriacontane-29-carbonyl]amino]-3-(1H-indol-3-yl)propanoyl]amino]-5-[[(1S)-1-carboxy-2-methylpropyl]amino]-5-oxopentanoic acid | |
---|---|---|
Source | PubChem | |
URL | https://pubchem.ncbi.nlm.nih.gov | |
Description | Data deposited in or computed by PubChem | |
InChI |
InChI=1S/C89H139N27O24S4/c1-43(2)67-82(135)101-40-64(119)102-53(18-12-30-97-87(91)92)74(127)113-68(44(3)4)83(136)109-59(36-47-22-24-49(118)25-23-47)77(130)107-58(20-14-32-99-89(95)96)85(138)116-33-15-21-63(116)81(134)111-62(80(133)108-60(37-48-39-100-52-17-11-10-16-50(48)52)78(131)104-55(26-27-65(120)121)75(128)114-69(45(5)6)86(139)140)42-144-143-41-61(79(132)105-57(29-35-142-9)76(129)112-67)110-72(125)54(19-13-31-98-88(93)94)103-73(126)56(28-34-141-8)106-84(137)70(46(7)117)115-71(124)51(90)38-66(122)123/h10-11,16-17,22-25,39,43-46,51,53-63,67-70,100,117-118H,12-15,18-21,26-38,40-42,90H2,1-9H3,(H,101,135)(H,102,119)(H,103,126)(H,104,131)(H,105,132)(H,106,137)(H,107,130)(H,108,133)(H,109,136)(H,110,125)(H,111,134)(H,112,129)(H,113,127)(H,114,128)(H,115,124)(H,120,121)(H,122,123)(H,139,140)(H4,91,92,97)(H4,93,94,98)(H4,95,96,99)/t46-,51+,53+,54+,55+,56+,57+,58+,59+,60+,61+,62+,63+,67+,68+,69+,70+/m1/s1 | |
Source | PubChem | |
URL | https://pubchem.ncbi.nlm.nih.gov | |
Description | Data deposited in or computed by PubChem | |
InChI Key |
ORRDHOMWDPJSNL-KHBOZDSRSA-N | |
Source | PubChem | |
URL | https://pubchem.ncbi.nlm.nih.gov | |
Description | Data deposited in or computed by PubChem | |
Canonical SMILES |
CC(C)C1C(=O)NCC(=O)NC(C(=O)NC(C(=O)NC(C(=O)NC(C(=O)N2CCCC2C(=O)NC(CSSCC(C(=O)NC(C(=O)N1)CCSC)NC(=O)C(CCCNC(=N)N)NC(=O)C(CCSC)NC(=O)C(C(C)O)NC(=O)C(CC(=O)O)N)C(=O)NC(CC3=CNC4=CC=CC=C43)C(=O)NC(CCC(=O)O)C(=O)NC(C(C)C)C(=O)O)CCCNC(=N)N)CC5=CC=C(C=C5)O)C(C)C)CCCNC(=N)N | |
Source | PubChem | |
URL | https://pubchem.ncbi.nlm.nih.gov | |
Description | Data deposited in or computed by PubChem | |
Isomeric SMILES |
C[C@H]([C@@H](C(=O)N[C@@H](CCSC)C(=O)N[C@@H](CCCNC(=N)N)C(=O)N[C@H]1CSSC[C@H](NC(=O)[C@@H]2CCCN2C(=O)[C@@H](NC(=O)[C@@H](NC(=O)[C@@H](NC(=O)[C@@H](NC(=O)CNC(=O)[C@@H](NC(=O)[C@@H](NC1=O)CCSC)C(C)C)CCCNC(=N)N)C(C)C)CC3=CC=C(C=C3)O)CCCNC(=N)N)C(=O)N[C@@H](CC4=CNC5=CC=CC=C54)C(=O)N[C@@H](CCC(=O)O)C(=O)N[C@@H](C(C)C)C(=O)O)NC(=O)[C@H](CC(=O)O)N)O | |
Source | PubChem | |
URL | https://pubchem.ncbi.nlm.nih.gov | |
Description | Data deposited in or computed by PubChem | |
Molecular Formula |
C89H139N27O24S4 | |
Source | PubChem | |
URL | https://pubchem.ncbi.nlm.nih.gov | |
Description | Data deposited in or computed by PubChem | |
DSSTOX Substance ID |
DTXSID40583241 | |
Record name | PUBCHEM_16167455 | |
Source | EPA DSSTox | |
URL | https://comptox.epa.gov/dashboard/DTXSID40583241 | |
Description | DSSTox provides a high quality public chemistry resource for supporting improved predictive toxicology. | |
Molecular Weight |
2099.5 g/mol | |
Source | PubChem | |
URL | https://pubchem.ncbi.nlm.nih.gov | |
Description | Data deposited in or computed by PubChem | |
CAS No. |
87218-84-6 | |
Record name | PUBCHEM_16167455 | |
Source | EPA DSSTox | |
URL | https://comptox.epa.gov/dashboard/DTXSID40583241 | |
Description | DSSTox provides a high quality public chemistry resource for supporting improved predictive toxicology. | |
Disclaimer and Information on In-Vitro Research Products
Please be aware that all articles and product information presented on BenchChem are intended solely for informational purposes. The products available for purchase on BenchChem are specifically designed for in-vitro studies, which are conducted outside of living organisms. In-vitro studies, derived from the Latin term "in glass," involve experiments performed in controlled laboratory settings using cells or tissues. It is important to note that these products are not categorized as medicines or drugs, and they have not received approval from the FDA for the prevention, treatment, or cure of any medical condition, ailment, or disease. We must emphasize that any form of bodily introduction of these products into humans or animals is strictly prohibited by law. It is essential to adhere to these guidelines to ensure compliance with legal and ethical standards in research and experimentation.