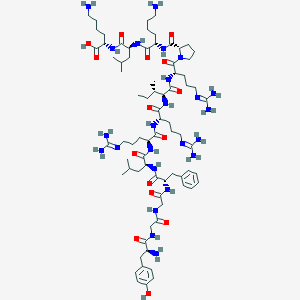
Dynorphin A (1-13)
Overview
Description
Dynorphin A1-13 is an endogenous opioid peptide that plays a crucial role in the body’s pain regulation and stress response systems. It is a part of the dynorphin family, which are peptides derived from the precursor protein prodynorphin. Dynorphin A1-13 specifically activates the kappa-opioid receptor, which is involved in modulating pain perception, mood, and stress .
Scientific Research Applications
Dynorphin A1-13 has a wide range of applications in scientific research:
Chemistry: Used to study peptide synthesis and modification techniques.
Biology: Investigated for its role in pain modulation, stress response, and neuroprotection.
Medicine: Explored as a potential therapeutic agent for pain management and mood disorders.
Industry: Utilized in the development of peptide-based drugs and diagnostic tools.
Mechanism of Action
Target of Action
Dynorphin A (1-13) is an endogenous opioid peptide that primarily targets the kappa-opioid receptor (KOR) . It also has some affinity for the mu and delta opioid receptors . The KOR is a G protein-coupled receptor that is widely distributed in the central nervous system and plays a crucial role in pain perception, mood regulation, and stress response .
Mode of Action
Dynorphin A (1-13) acts as a full agonist at the KOR . Upon binding to the KOR, it triggers a series of intracellular events, including the inhibition of adenylate cyclase, the activation of potassium channels, and the inhibition of calcium channels . This leads to hyperpolarization of the neuron and a decrease in neuronal excitability .
Biochemical Pathways
The activation of KOR by Dynorphin A (1-13) can modulate several biochemical pathways. For instance, it can inhibit the release of neurotransmitters such as gamma-aminobutyric acid (GABA) and glutamate , thereby modulating synaptic transmission . Furthermore, Dynorphin A (1-13) is metabolized by various enzymes, including carboxypeptidases and aminopeptidases, into several metabolites, such as Dyn A1-12, A2-12, A4-12, and A4-8 .
Pharmacokinetics
Dynorphin A (1-13) has a short half-life in human plasma and blood, less than one minute at 37°C . Its major plasma metabolites, including Dyn A1-12, A2-12, A4-12, and A4-8, have half-lives ranging between 0.5 and 4 minutes . These pharmacokinetic properties suggest that Dynorphin A (1-13) is rapidly metabolized and cleared from the body .
Result of Action
The activation of KOR by Dynorphin A (1-13) can have various effects at the molecular and cellular levels. For instance, it can modulate neuronal excitability, inhibit neurotransmitter release, and alter synaptic transmission . In addition, it has been suggested that Dynorphin A (1-13) can attenuate the impairment of memory processes through the mediation of KOR .
Action Environment
The action of Dynorphin A (1-13) can be influenced by various environmental factors. For example, its activity can be modulated by the presence of other neurotransmitters, the state of the neuronal membrane, and the intracellular environment . Furthermore, its action can be affected by the presence of other opioid peptides and the overall state of the opioid system .
Safety and Hazards
Biochemical Analysis
Biochemical Properties
Dynorphin A (1-13) interacts with several enzymes, proteins, and other biomolecules, influencing various biochemical reactions. It primarily binds to kappa-opioid receptors, which are G-protein-coupled receptors involved in pain modulation and stress response. The interaction between Dynorphin A (1-13) and kappa-opioid receptors leads to the inhibition of adenylate cyclase, reducing cyclic adenosine monophosphate levels and subsequently decreasing neurotransmitter release. Additionally, Dynorphin A (1-13) is metabolized by carboxypeptidases and aminopeptidases, resulting in various cleavage products such as Dynorphin A (1-12) and Dynorphin A (2-13) .
Cellular Effects
Dynorphin A (1-13) exerts significant effects on various cell types and cellular processes. In neurons, it modulates neurotransmitter release, influencing pain perception and emotional responses. Dynorphin A (1-13) also affects glial cells, contributing to neuroinflammation and neuroprotection. It influences cell signaling pathways, such as the mitogen-activated protein kinase pathway, and alters gene expression, impacting cellular metabolism and function .
Molecular Mechanism
The mechanism of action of Dynorphin A (1-13) involves binding to kappa-opioid receptors, leading to the activation of G-proteins and subsequent inhibition of adenylate cyclase. This results in decreased cyclic adenosine monophosphate levels and reduced neurotransmitter release. Dynorphin A (1-13) also interacts with other receptors, such as N-methyl-D-aspartate receptors, modulating their activity and contributing to its analgesic and neuroprotective effects. Additionally, Dynorphin A (1-13) influences gene expression by regulating transcription factors and epigenetic modifications .
Temporal Effects in Laboratory Settings
In laboratory settings, the effects of Dynorphin A (1-13) change over time due to its stability and degradation. Dynorphin A (1-13) has a short half-life in plasma, with major metabolites having half-lives ranging from 0.5 to 4 minutes . In cerebrospinal fluid, the half-life of Dynorphin A (1-13) is approximately 2.5 hours . Long-term effects on cellular function include alterations in neurotransmitter release, gene expression, and cellular metabolism, which can be observed in both in vitro and in vivo studies .
Dosage Effects in Animal Models
The effects of Dynorphin A (1-13) vary with different dosages in animal models. At low doses, Dynorphin A (1-13) exhibits analgesic and neuroprotective effects, while higher doses can lead to adverse effects such as motor impairment and neurotoxicity. Threshold effects are observed, with specific dosages required to achieve desired outcomes without causing toxicity .
Metabolic Pathways
Dynorphin A (1-13) is involved in several metabolic pathways, primarily being metabolized by carboxypeptidases and aminopeptidases. These enzymes cleave Dynorphin A (1-13) into various fragments, such as Dynorphin A (1-12) and Dynorphin A (2-13). The metabolic flux and levels of metabolites are influenced by the activity of these enzymes and the presence of enzyme inhibitors .
Transport and Distribution
Dynorphin A (1-13) is transported and distributed within cells and tissues through various mechanisms. It interacts with transporters and binding proteins, influencing its localization and accumulation. Dynorphin A (1-13) can cross the blood-brain barrier, allowing it to exert its effects on the central nervous system. Its distribution is also affected by its binding to kappa-opioid receptors and other biomolecules .
Subcellular Localization
The subcellular localization of Dynorphin A (1-13) is crucial for its activity and function. It is primarily localized in synaptic vesicles and secretory granules, where it is released in response to neuronal activity. Post-translational modifications, such as phosphorylation, can influence its targeting to specific compartments or organelles, affecting its interactions with receptors and other biomolecules .
Preparation Methods
Synthetic Routes and Reaction Conditions: Dynorphin A1-13 can be synthesized using solid-phase peptide synthesis (SPPS), a method commonly employed for the production of peptides. The process involves the sequential addition of amino acids to a growing peptide chain anchored to a solid resin. The amino acids are protected by specific groups to prevent unwanted reactions. After the assembly of the peptide chain, the protecting groups are removed, and the peptide is cleaved from the resin .
Industrial Production Methods: Industrial production of Dynorphin A1-13 follows similar principles as laboratory synthesis but on a larger scale. Automated peptide synthesizers are used to enhance efficiency and yield. The process involves rigorous purification steps, including high-performance liquid chromatography (HPLC), to ensure the purity of the final product .
Chemical Reactions Analysis
Types of Reactions: Dynorphin A1-13 undergoes various enzymatic reactions, primarily involving proteolytic cleavage. These reactions include:
Oxidation: Dynorphin A1-13 can be oxidized, leading to the formation of disulfide bonds between cysteine residues.
Reduction: Reduction reactions can break disulfide bonds, reverting the peptide to its reduced form.
Common Reagents and Conditions:
Oxidation: Hydrogen peroxide or other oxidizing agents.
Reduction: Dithiothreitol (DTT) or beta-mercaptoethanol.
Substitution: Site-directed mutagenesis using specific primers and DNA polymerase.
Major Products Formed:
Oxidation: Formation of disulfide-bonded peptides.
Reduction: Reduced form of the peptide.
Substitution: Mutant peptides with altered amino acid sequences.
Comparison with Similar Compounds
Dynorphin A1-8: A truncated form of Dynorphin A1-13 with similar but less potent effects.
Beta-Endorphin: Another endogenous opioid peptide that primarily activates the mu-opioid receptor.
Enkephalins: Short peptides that activate delta-opioid receptors.
Uniqueness of Dynorphin A1-13: Dynorphin A1-13 is unique due to its high affinity and selectivity for the kappa-opioid receptor. This specificity allows it to modulate pain and stress responses more effectively than other opioid peptides. Additionally, its ability to induce dysphoria and aversion distinguishes it from other opioid peptides that typically produce euphoria .
Properties
IUPAC Name |
(2S)-6-amino-2-[[(2S)-2-[[(2S)-6-amino-2-[[(2S)-1-[(2S)-2-[[(2S,3S)-2-[[(2S)-2-[[(2S)-2-[[(2S)-2-[[(2S)-2-[[2-[[2-[[(2S)-2-amino-3-(4-hydroxyphenyl)propanoyl]amino]acetyl]amino]acetyl]amino]-3-phenylpropanoyl]amino]-4-methylpentanoyl]amino]-5-(diaminomethylideneamino)pentanoyl]amino]-5-(diaminomethylideneamino)pentanoyl]amino]-3-methylpentanoyl]amino]-5-(diaminomethylideneamino)pentanoyl]pyrrolidine-2-carbonyl]amino]hexanoyl]amino]-4-methylpentanoyl]amino]hexanoic acid | |
---|---|---|
Source | PubChem | |
URL | https://pubchem.ncbi.nlm.nih.gov | |
Description | Data deposited in or computed by PubChem | |
InChI |
InChI=1S/C75H126N24O15/c1-7-45(6)61(70(111)94-53(25-17-35-87-75(83)84)71(112)99-36-18-26-58(99)69(110)93-50(21-11-13-31-76)64(105)96-56(38-44(4)5)67(108)95-54(72(113)114)22-12-14-32-77)98-65(106)52(24-16-34-86-74(81)82)91-63(104)51(23-15-33-85-73(79)80)92-66(107)55(37-43(2)3)97-68(109)57(40-46-19-9-8-10-20-46)90-60(102)42-88-59(101)41-89-62(103)49(78)39-47-27-29-48(100)30-28-47/h8-10,19-20,27-30,43-45,49-58,61,100H,7,11-18,21-26,31-42,76-78H2,1-6H3,(H,88,101)(H,89,103)(H,90,102)(H,91,104)(H,92,107)(H,93,110)(H,94,111)(H,95,108)(H,96,105)(H,97,109)(H,98,106)(H,113,114)(H4,79,80,85)(H4,81,82,86)(H4,83,84,87)/t45-,49-,50-,51-,52-,53-,54-,55-,56-,57-,58-,61-/m0/s1 | |
Source | PubChem | |
URL | https://pubchem.ncbi.nlm.nih.gov | |
Description | Data deposited in or computed by PubChem | |
InChI Key |
OVVIBUHLQIYUEU-IWIISZHXSA-N | |
Source | PubChem | |
URL | https://pubchem.ncbi.nlm.nih.gov | |
Description | Data deposited in or computed by PubChem | |
Canonical SMILES |
CCC(C)C(C(=O)NC(CCCN=C(N)N)C(=O)N1CCCC1C(=O)NC(CCCCN)C(=O)NC(CC(C)C)C(=O)NC(CCCCN)C(=O)O)NC(=O)C(CCCN=C(N)N)NC(=O)C(CCCN=C(N)N)NC(=O)C(CC(C)C)NC(=O)C(CC2=CC=CC=C2)NC(=O)CNC(=O)CNC(=O)C(CC3=CC=C(C=C3)O)N | |
Source | PubChem | |
URL | https://pubchem.ncbi.nlm.nih.gov | |
Description | Data deposited in or computed by PubChem | |
Isomeric SMILES |
CC[C@H](C)[C@@H](C(=O)N[C@@H](CCCN=C(N)N)C(=O)N1CCC[C@H]1C(=O)N[C@@H](CCCCN)C(=O)N[C@@H](CC(C)C)C(=O)N[C@@H](CCCCN)C(=O)O)NC(=O)[C@H](CCCN=C(N)N)NC(=O)[C@H](CCCN=C(N)N)NC(=O)[C@H](CC(C)C)NC(=O)[C@H](CC2=CC=CC=C2)NC(=O)CNC(=O)CNC(=O)[C@H](CC3=CC=C(C=C3)O)N | |
Source | PubChem | |
URL | https://pubchem.ncbi.nlm.nih.gov | |
Description | Data deposited in or computed by PubChem | |
Molecular Formula |
C75H126N24O15 | |
Source | PubChem | |
URL | https://pubchem.ncbi.nlm.nih.gov | |
Description | Data deposited in or computed by PubChem | |
Molecular Weight |
1604.0 g/mol | |
Source | PubChem | |
URL | https://pubchem.ncbi.nlm.nih.gov | |
Description | Data deposited in or computed by PubChem | |
CAS No. |
72957-38-1 | |
Record name | Dynorphin (1-13) | |
Source | ChemIDplus | |
URL | https://pubchem.ncbi.nlm.nih.gov/substance/?source=chemidplus&sourceid=0072957381 | |
Description | ChemIDplus is a free, web search system that provides access to the structure and nomenclature authority files used for the identification of chemical substances cited in National Library of Medicine (NLM) databases, including the TOXNET system. | |
Record name | DYNORPHIN A 1-13 | |
Source | FDA Global Substance Registration System (GSRS) | |
URL | https://gsrs.ncats.nih.gov/ginas/app/beta/substances/VFC23V742Z | |
Description | The FDA Global Substance Registration System (GSRS) enables the efficient and accurate exchange of information on what substances are in regulated products. Instead of relying on names, which vary across regulatory domains, countries, and regions, the GSRS knowledge base makes it possible for substances to be defined by standardized, scientific descriptions. | |
Explanation | Unless otherwise noted, the contents of the FDA website (www.fda.gov), both text and graphics, are not copyrighted. They are in the public domain and may be republished, reprinted and otherwise used freely by anyone without the need to obtain permission from FDA. Credit to the U.S. Food and Drug Administration as the source is appreciated but not required. | |
Retrosynthesis Analysis
AI-Powered Synthesis Planning: Our tool employs the Template_relevance Pistachio, Template_relevance Bkms_metabolic, Template_relevance Pistachio_ringbreaker, Template_relevance Reaxys, Template_relevance Reaxys_biocatalysis model, leveraging a vast database of chemical reactions to predict feasible synthetic routes.
One-Step Synthesis Focus: Specifically designed for one-step synthesis, it provides concise and direct routes for your target compounds, streamlining the synthesis process.
Accurate Predictions: Utilizing the extensive PISTACHIO, BKMS_METABOLIC, PISTACHIO_RINGBREAKER, REAXYS, REAXYS_BIOCATALYSIS database, our tool offers high-accuracy predictions, reflecting the latest in chemical research and data.
Strategy Settings
Precursor scoring | Relevance Heuristic |
---|---|
Min. plausibility | 0.01 |
Model | Template_relevance |
Template Set | Pistachio/Bkms_metabolic/Pistachio_ringbreaker/Reaxys/Reaxys_biocatalysis |
Top-N result to add to graph | 6 |
Feasible Synthetic Routes
Q1: What is the primary target of Dynorphin A (1-13)?
A1: Dynorphin A (1-13) primarily targets the kappa-opioid receptor (KOR) [, , , , , , ].
Q2: What are the downstream effects of Dynorphin A (1-13) binding to KORs?
A2: Dynorphin A (1-13) binding to KORs elicits a variety of downstream effects, including: - Inhibition of adenylyl cyclase activity []- Modulation of calcium and potassium channels [, ]- Inhibition of neurotransmitter release, including acetylcholine, dopamine, and GABA [, , , , ]
Q3: Does Dynorphin A (1-13) interact with other opioid receptors?
A3: While Dynorphin A (1-13) exhibits high affinity for KORs, it can also interact with mu-opioid receptors (MORs) and delta-opioid receptors (DORs), albeit with lower affinity [, , , ].
Q4: Does Dynorphin A (1-13) exhibit both pre- and post-synaptic effects?
A4: Yes, Dynorphin A (1-13) demonstrates both pre- and postsynaptic effects. It can hyperpolarize dopamine neurons postsynaptically and suppress dopamine release through actions at presynaptic release sites [].
Q5: What is the molecular formula and weight of Dynorphin A (1-13)?
A5: The molecular formula of Dynorphin A (1-13) is C70H109N21O16, and its molecular weight is 1496.7 g/mol [].
Q6: Is there spectroscopic data available for Dynorphin A (1-13)?
A6: Research has utilized techniques like mass spectrometry to study Dynorphin A (1-13). For instance, hydrogen-deuterium exchange coupled with mass spectrometry was employed to analyze conformational changes induced by trifluoroethanol [].
Q7: How do structural modifications affect the activity of Dynorphin A (1-13)?
A7: Structural modifications significantly impact the activity of Dynorphin A (1-13). For example:
- Dynorphin A (3-13): This fragment, lacking the N-terminal tyrosine, exhibits non-opioid anticonvulsant effects but does not possess the opioid antagonist properties of Dynorphin A (1-13) [, ].
- Dynorphin A (13-17): This C-terminal fragment exhibits neurotoxic effects, suggesting the presence of intrinsic neurotoxicity within this region of Dynorphin A [].
- Arodyn: This synthetic analog of Dynorphin A (1-11) with a modified N-terminus acts as a potent and selective KOR antagonist [].
Q8: Are there any Dynorphin A (1-13) analogs designed for enhanced stability or specific targeting?
A8: CJC-1008 is a chemically modified version of Dynorphin A (1-13) designed to covalently bind to human serum albumin, thereby prolonging its duration of action in vivo [].
Q9: How stable is Dynorphin A (1-13) in biological systems?
A9: Dynorphin A (1-13) is susceptible to rapid enzymatic degradation in vivo, limiting its duration of action. Studies have shown that peptidase inhibitors can significantly enhance the paralytic effects of Dynorphin A (1-13), indicating the role of peptidases in its degradation [].
Q10: What in vitro models are used to study the effects of Dynorphin A (1-13)?
A10: Researchers commonly use isolated tissue preparations, such as guinea pig colon circular muscle [], and primary neuronal cultures [, ] to study the effects of Dynorphin A (1-13) in vitro.
Q11: What in vivo models are used to investigate Dynorphin A (1-13)?
A11: Several animal models are employed to study Dynorphin A (1-13) in vivo, including:- Pain Models: Formalin-induced flinching in rats is used to investigate the role of Dynorphin A (1-13) in nociception [].- Stress Models: Immobilization stress and swimming stress models in rodents help understand the influence of Dynorphin A (1-13) on stress responses and potential protective effects [, , , ].- Learning and Memory Impairment: Rodent models of learning and memory impairment induced by cholinergic antagonists or NMDA receptor blockers are utilized to investigate the potential role of Dynorphin A (1-13) in cognitive function [, ].
Disclaimer and Information on In-Vitro Research Products
Please be aware that all articles and product information presented on BenchChem are intended solely for informational purposes. The products available for purchase on BenchChem are specifically designed for in-vitro studies, which are conducted outside of living organisms. In-vitro studies, derived from the Latin term "in glass," involve experiments performed in controlled laboratory settings using cells or tissues. It is important to note that these products are not categorized as medicines or drugs, and they have not received approval from the FDA for the prevention, treatment, or cure of any medical condition, ailment, or disease. We must emphasize that any form of bodily introduction of these products into humans or animals is strictly prohibited by law. It is essential to adhere to these guidelines to ensure compliance with legal and ethical standards in research and experimentation.