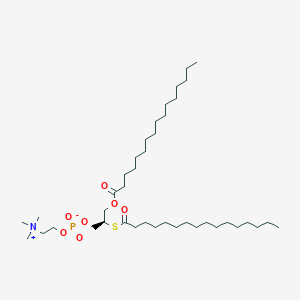
Palmitoyl Thio-PC
Overview
Description
Palmitoyl Thio-PC (C40H80NO7PS, molecular weight 750.114 g/mol) is a phosphatidylcholine derivative distinguished by a thioester group at the sn-2 position of the glycerol backbone . Its structure comprises a palmitoyl fatty acid chain (C16:0) at the sn-1 position and a sulfur-containing thioester at sn-2, replacing the conventional ester bond . This modification enhances its reactivity, particularly under oxidative conditions, enabling applications in studying membrane dynamics, lipid-protein interactions, and phospholipase A2 (PLA2) activity .
This compound serves as a chromogenic substrate for PLA2 enzymes. Hydrolysis of the thioester bond releases a free thiol group, which reacts with 5,5'-dithiobis-(2-nitrobenzoic acid) (DTNB, Ellman's reagent) to generate a yellow-colored product (absorbance at 412 nm), facilitating quantitative enzyme activity assays .
Preparation Methods
Synthetic Routes and Reaction Conditions
Palmitoyl thio-phosphatidylcholine can be synthesized through a chiral synthesis process. The reaction conditions typically involve the use of organic solvents such as ethanol and phosphate-buffered saline at a pH of 7.2 .
Industrial Production Methods
Industrial production of palmitoyl thio-phosphatidylcholine involves large-scale synthesis using similar methods as described above. The process is optimized for high yield and purity, ensuring that the final product meets the required standards for biochemical assays .
Chemical Reactions Analysis
Types of Reactions
Palmitoyl thio-phosphatidylcholine primarily undergoes hydrolysis reactions. The hydrolysis of the thioester bond by phospholipase A2 yields a free thiol, which can react with Ellman’s reagent (5,5’-dithiobis-(2-nitrobenzoic acid)) to produce a bright yellow product .
Common Reagents and Conditions
Reagents: Phospholipase A2, Ellman’s reagent
Conditions: Aqueous buffer systems, typically at neutral pH
Major Products
The major product formed from the hydrolysis of palmitoyl thio-phosphatidylcholine is a free thiol, which reacts with Ellman’s reagent to form a yellow-colored product with an absorbance maximum at 412 nm .
Scientific Research Applications
Role in Neurobiology
Palmitoyl Thio-PC serves as a substrate for palmitoyl protein thioesterase 1 (PPT1), an enzyme crucial for the depalmitoylation of proteins involved in synaptic function and neurotransmission. Recent studies have identified over 600 palmitoylated proteins in the mammalian brain, highlighting the importance of palmitoylation in neuronal health and signaling pathways. The loss-of-function mutations in PPT1 lead to neuronal ceroid lipofuscinosis (NCL), a neurodegenerative disease characterized by synaptic dysfunction and mitochondrial impairment .
Case Study: PPT1 Substrates Identification
A systematic proteomic study utilized Acyl Resin-Assisted Capture (Acyl RAC) combined with mass spectrometry to identify novel PPT1 substrates. The findings revealed that PPT1 is involved in various cellular processes, including:
- Synaptic adhesion
- Ion transport
- Endo/exocytic processes
Out of 204 putative substrates, 138 were validated, underscoring the enzyme's role in maintaining synaptic integrity and function .
Cancer Research Applications
PPT1 has been implicated in various cancers, particularly cholangiocarcinoma and esophageal cancers. Inhibitors of PPT1 have shown promise in inducing cell death in tumor cells, making it a viable target for anticancer drug development. For instance, compounds like orlistat and palmostatin B have been identified as potent inhibitors of PPT1, demonstrating significant efficacy in neuroblastoma cell lines .
Table: Inhibitors of PPT1 and Their Potency
Inhibitor | IC50 (nM) | Cancer Type |
---|---|---|
Orlistat | 178.8 | Various |
Palmostatin B | 11.8 | Neuroblastoma |
These findings suggest that targeting PPT1 could enhance therapeutic strategies against certain cancers by promoting apoptosis in malignant cells .
Enzymatic Studies
This compound's thioester linkage enhances its reactivity with enzymes such as phospholipase A2 (PLA2). This property is exploited in biochemical assays to study enzyme-substrate interactions and the mechanisms of lipid metabolism. The compound's unique structure allows researchers to investigate how palmitoylation affects protein function and stability, particularly concerning membrane-associated proteins .
Mechanism of Action
The mechanism of action of palmitoyl thio-phosphatidylcholine involves its hydrolysis by phospholipase A2. The enzyme cleaves the thioester bond at the sn-2 position, releasing a free thiol. This free thiol can then react with Ellman’s reagent to produce a yellow-colored product, which can be measured spectrophotometrically .
Comparison with Similar Compounds
Structural and Functional Differences
Table 1: Key Structural and Functional Features of Palmitoyl Thio-PC and Analogous Compounds
Key Observations:
Thioester vs. Oxyester : The thioester bond in this compound increases its susceptibility to oxidation and disulfide bond formation compared to oxyester analogs like PC 14:0/14:0. This property is exploited in redox-sensitive membrane studies .
Fatty Acid Chain Length: this compound’s long-chain palmitoyl group mimics natural phospholipids, whereas shorter chains (e.g., heptanoyl) improve solubility but reduce biological relevance .
Enzyme Specificity: Arachidonoyl Thio-PC is preferred for cPLA2 due to its polyunsaturated chain, while this compound is optimal for sPLA2 in mixed micelle systems .
Biochemical and Pharmacological Roles
Key Observations:
Cancer Relevance : this compound and PC 38:6 are both upregulated in aggressive cancers, but this compound uniquely contributes to membrane biogenesis via its thioester-mediated reactivity .
Membrane Effects : Unlike Palmitoyl Carnitine, which increases hydrophilic drug permeability by disordering lipid bilayers , this compound facilitates reversible membrane crosslinking, aiding structural studies .
Analytical and Commercial Considerations
- Sensitivity in Assays: this compound’s chromogenic response (ε412 = 13,600 M⁻¹cm⁻¹) is superior to non-thiolated analogs, enabling low-detection limits (~nmol/min/mg protein) .
- Commercial Availability: this compound is sold at $184/mg (Santa Cruz Biotechnology), whereas Arachidonoyl Thio-PC costs ~$500/mg due to complex synthesis .
Biological Activity
Palmitoyl Thio-PC (Palmitoyl Thio-Phosphatidylcholine) is a phospholipid derivative that has garnered attention for its potential biological activities, particularly in the context of cellular signaling, membrane dynamics, and therapeutic applications. This article reviews the biological activity of this compound, highlighting its mechanisms of action, therapeutic implications, and relevant case studies.
Overview of this compound
This compound is a modified form of phosphatidylcholine where a palmitoyl group is attached via a thioester bond. This modification affects its interaction with biological membranes and proteins, influencing various cellular processes. The compound is particularly relevant in studies focusing on palmitoylation—a post-translational modification that regulates protein function and localization.
-
Protein Palmitoylation :
- Palmitoylation is crucial for the membrane localization and function of many proteins. This compound can act as a substrate for palmitoyl-protein thioesterases (PPTs), which are enzymes that remove palmitate from proteins, thereby regulating their activity and stability.
- PPT1, a key enzyme involved in this process, has been implicated in neurodegenerative diseases and cancer. Inhibition of PPT1 can lead to increased levels of palmitoylated proteins, potentially enhancing their function or altering signaling pathways associated with cell survival and proliferation .
-
Cell Membrane Dynamics :
- The incorporation of this compound into cell membranes can affect membrane fluidity and permeability. Its thioester bond may enhance interactions with membrane proteins, influencing cellular signaling pathways.
- Studies have shown that modifications in membrane composition can alter the behavior of signaling molecules such as G-proteins and receptor tyrosine kinases, which are critical for various cellular responses .
Therapeutic Implications
This compound has potential therapeutic applications in several areas:
-
Cancer Therapy :
- Research indicates that inhibiting PPT1 can enhance the efficacy of chemotherapeutic agents by promoting apoptosis in tumor cells. For instance, compounds like orlistat and palmostatin B have been identified as potent inhibitors of PPT1, leading to increased cell death in cancer models .
- By modulating the activity of PPT1 through the use of this compound or similar compounds, it may be possible to develop new strategies for cancer treatment.
-
Neurodegenerative Diseases :
- Given its role in regulating protein stability and function through palmitoylation, this compound may offer therapeutic benefits in conditions like infantile neuronal ceroid lipofuscinosis (CLN1 disease). Enhancing PPT1 activity could potentially alleviate symptoms associated with lysosomal storage disorders .
Case Studies
Several studies have explored the effects of this compound and related compounds:
- Study on PPT1 Inhibition :
Compound | IC50 (nM) | Effect on PPT1 Activity |
---|---|---|
Orlistat | 178.8 | Poor inhibition |
Palmostatin B | 11.8 | Potent inhibition |
CS38 | Not specified | Enhanced activity in mutants |
Q & A
Basic Research Questions
Q. How can Palmitoyl Thio-PC be reliably identified and quantified in biological samples?
- Methodological Answer : Use liquid chromatography-tandem mass spectrometry (LC-MS/MS) with optimized ionization parameters for phosphatidylcholine derivatives. Validate the method using internal standards (e.g., deuterated analogs) to account for matrix effects. For quantitative analysis, ensure calibration curves cover physiological and pathological concentration ranges observed in cancer studies (e.g., 0.1–50 μM) .
Q. What is the primary biological role of this compound in cellular membranes?
- Methodological Answer : this compound contributes to membrane biogenesis by integrating into lipid bilayers, enhancing membrane fluidity, and supporting rapid cell proliferation. Its thioester bond may influence oxidative stability compared to native phosphatidylcholines. Experimental validation involves comparative lipidomics in cancer cell lines (e.g., using siRNA knockdown of lipid synthesis enzymes) .
Q. What analytical techniques are suitable for detecting this compound in metabolomic studies?
- Methodological Answer : Combine high-resolution mass spectrometry (HRMS) with collision-induced dissociation (CID) to distinguish this compound from isobaric lipids. Pair this with nuclear magnetic resonance (NMR) to confirm structural specificity, particularly the thioester linkage. Statistical validation should follow protocols for metabolomic data normalization (e.g., variance-stabilizing transformation) .
Advanced Research Questions
Q. How can researchers address contradictory findings regarding this compound’s accumulation in different cancer grades?
- Methodological Answer : Stratify patient cohorts by tumor grade and histological subtype to control for heterogeneity. Use multivariate regression models to adjust for confounding variables (e.g., lipid metabolism enzymes, dietary influences). Replicate findings in in vitro models with controlled lipid microenvironments to isolate mechanistic drivers .
Q. What experimental designs are optimal for studying this compound’s interaction with lipid-metabolizing enzymes (e.g., phospholipase A2)?
- Methodological Answer : Employ kinetic assays with purified enzymes and synthetic this compound substrates. Monitor reaction products via thin-layer chromatography (TLC) or fluorometric assays. Compare kinetic parameters (e.g., , ) to native phosphatidylcholines to assess enzymatic specificity. Include negative controls with thioesterase inhibitors to rule off-target effects .
Q. How can this compound’s role in cancer proliferation be disentangled from other phosphatidylcholines?
- Methodological Answer : Use isotope tracing (e.g., -labeled palmitate) to track its incorporation into membrane lipids versus signaling metabolites. Combine this with CRISPR-Cas9 knockout models of key lipid transporters (e.g., MFSD2A) to evaluate functional redundancy among phosphatidylcholines. Validate findings using lipid bilayer permeability assays .
Q. What statistical approaches are recommended for analyzing dose-dependent effects of this compound in cellular assays?
- Methodological Answer : Apply nonlinear regression models (e.g., sigmoidal dose-response curves) to estimate EC values. Use bootstrapping to assess confidence intervals, especially with small sample sizes. For multi-omics integration, apply pathway enrichment analysis (e.g., MetaboAnalyst) to link lipidomic changes to transcriptomic or proteomic data .
Q. Methodological Challenges and Solutions
Q. How should researchers handle the oxidative instability of this compound during experimental workflows?
- Methodological Answer : Store samples under inert gas (e.g., argon) and add antioxidants (e.g., butylated hydroxytoluene) to lipid extraction buffers. Validate stability via time-course LC-MS measurements. For in vivo studies, use acute exposure models to minimize degradation artifacts .
Q. What strategies mitigate batch-to-batch variability in synthetic this compound preparations?
- Methodological Answer : Implement rigorous quality control (QC) protocols:
- Purity : Verify via HPLC-ELSD (>95% purity).
- Structural fidelity : Confirm thioester linkage via -NMR (δ 2.85–3.10 ppm for thioester protons).
- Functional consistency : Test each batch in a standardized membrane fluidity assay .
Q. How can this compound’s metabolic crosstalk with acyl-CoA pathways be systematically mapped?
- Methodological Answer : Use stable isotope-resolved lipidomics (-glucose or -glutamine tracing) to track carbon flux into this compound. Integrate with transcriptomic data (e.g., RNA-seq of ACSL and CPT1 genes) to identify regulatory nodes. Computational modeling (e.g., constraint-based reconstruction of lipid networks) can predict rate-limiting steps .
Properties
IUPAC Name |
[(2R)-3-hexadecanoyloxy-2-hexadecanoylsulfanylpropyl] 2-(trimethylazaniumyl)ethyl phosphate | |
---|---|---|
Source | PubChem | |
URL | https://pubchem.ncbi.nlm.nih.gov | |
Description | Data deposited in or computed by PubChem | |
InChI |
InChI=1S/C40H80NO7PS/c1-6-8-10-12-14-16-18-20-22-24-26-28-30-32-39(42)46-36-38(37-48-49(44,45)47-35-34-41(3,4)5)50-40(43)33-31-29-27-25-23-21-19-17-15-13-11-9-7-2/h38H,6-37H2,1-5H3/t38-/m1/s1 | |
Source | PubChem | |
URL | https://pubchem.ncbi.nlm.nih.gov | |
Description | Data deposited in or computed by PubChem | |
InChI Key |
CJNCGLSWHABGOT-KXQOOQHDSA-N | |
Source | PubChem | |
URL | https://pubchem.ncbi.nlm.nih.gov | |
Description | Data deposited in or computed by PubChem | |
Canonical SMILES |
CCCCCCCCCCCCCCCC(=O)OCC(COP(=O)([O-])OCC[N+](C)(C)C)SC(=O)CCCCCCCCCCCCCCC | |
Source | PubChem | |
URL | https://pubchem.ncbi.nlm.nih.gov | |
Description | Data deposited in or computed by PubChem | |
Isomeric SMILES |
CCCCCCCCCCCCCCCC(=O)OC[C@H](COP(=O)([O-])OCC[N+](C)(C)C)SC(=O)CCCCCCCCCCCCCCC | |
Source | PubChem | |
URL | https://pubchem.ncbi.nlm.nih.gov | |
Description | Data deposited in or computed by PubChem | |
Molecular Formula |
C40H80NO7PS | |
Source | PubChem | |
URL | https://pubchem.ncbi.nlm.nih.gov | |
Description | Data deposited in or computed by PubChem | |
DSSTOX Substance ID |
DTXSID50921231 | |
Record name | 3-(Hexadecanoyloxy)-2-(hexadecanoylsulfanyl)propyl 2-(trimethylazaniumyl)ethyl phosphate | |
Source | EPA DSSTox | |
URL | https://comptox.epa.gov/dashboard/DTXSID50921231 | |
Description | DSSTox provides a high quality public chemistry resource for supporting improved predictive toxicology. | |
Molecular Weight |
750.1 g/mol | |
Source | PubChem | |
URL | https://pubchem.ncbi.nlm.nih.gov | |
Description | Data deposited in or computed by PubChem | |
CAS No. |
113881-60-0 | |
Record name | 1-Palmitoyl-2-thiopalmitoyl phosphatidylcholine | |
Source | ChemIDplus | |
URL | https://pubchem.ncbi.nlm.nih.gov/substance/?source=chemidplus&sourceid=0113881600 | |
Description | ChemIDplus is a free, web search system that provides access to the structure and nomenclature authority files used for the identification of chemical substances cited in National Library of Medicine (NLM) databases, including the TOXNET system. | |
Record name | 3-(Hexadecanoyloxy)-2-(hexadecanoylsulfanyl)propyl 2-(trimethylazaniumyl)ethyl phosphate | |
Source | EPA DSSTox | |
URL | https://comptox.epa.gov/dashboard/DTXSID50921231 | |
Description | DSSTox provides a high quality public chemistry resource for supporting improved predictive toxicology. | |
Retrosynthesis Analysis
AI-Powered Synthesis Planning: Our tool employs the Template_relevance Pistachio, Template_relevance Bkms_metabolic, Template_relevance Pistachio_ringbreaker, Template_relevance Reaxys, Template_relevance Reaxys_biocatalysis model, leveraging a vast database of chemical reactions to predict feasible synthetic routes.
One-Step Synthesis Focus: Specifically designed for one-step synthesis, it provides concise and direct routes for your target compounds, streamlining the synthesis process.
Accurate Predictions: Utilizing the extensive PISTACHIO, BKMS_METABOLIC, PISTACHIO_RINGBREAKER, REAXYS, REAXYS_BIOCATALYSIS database, our tool offers high-accuracy predictions, reflecting the latest in chemical research and data.
Strategy Settings
Precursor scoring | Relevance Heuristic |
---|---|
Min. plausibility | 0.01 |
Model | Template_relevance |
Template Set | Pistachio/Bkms_metabolic/Pistachio_ringbreaker/Reaxys/Reaxys_biocatalysis |
Top-N result to add to graph | 6 |
Feasible Synthetic Routes
Disclaimer and Information on In-Vitro Research Products
Please be aware that all articles and product information presented on BenchChem are intended solely for informational purposes. The products available for purchase on BenchChem are specifically designed for in-vitro studies, which are conducted outside of living organisms. In-vitro studies, derived from the Latin term "in glass," involve experiments performed in controlled laboratory settings using cells or tissues. It is important to note that these products are not categorized as medicines or drugs, and they have not received approval from the FDA for the prevention, treatment, or cure of any medical condition, ailment, or disease. We must emphasize that any form of bodily introduction of these products into humans or animals is strictly prohibited by law. It is essential to adhere to these guidelines to ensure compliance with legal and ethical standards in research and experimentation.