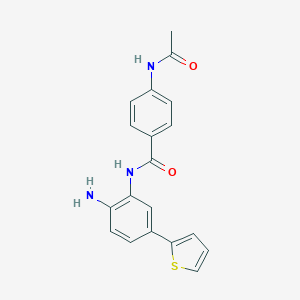
BRD-6929
Overview
Description
JQ12 is a chemical compound known for its selective inhibition of histone deacetylase 1 and histone deacetylase 2. Histone deacetylases are enzymes that remove acetyl groups from histone proteins, leading to chromatin condensation and transcriptional repression. JQ12 has been studied extensively for its potential therapeutic applications, particularly in cancer treatment, due to its ability to modulate gene expression through epigenetic mechanisms .
Mechanism of Action
Mode of Action
BRD-6929 interacts with its targets, HDAC1 and HDAC2, by binding to them with high affinity . The Ki values, which represent the binding affinity, are 0.2 nM for HDAC1 and 1.5 nM for HDAC2 . This interaction results in the inhibition of these enzymes, thereby affecting the acetylation status of histones and other proteins .
Biochemical Pathways
The inhibition of HDAC1 and HDAC2 by this compound affects the epigenetic regulation of gene expression . This can alter the transcription of various genes, including oncogenes and tumor suppressor genes . Moreover, this compound can induce a dose-dependent acetylation of H4K12ac in cultured neurons .
Pharmacokinetics
It is noted that this compound is brain-penetrant , which suggests that it can cross the blood-brain barrier and exert its effects in the brain.
Result of Action
It causes an increase in H4K12 acetylation in brain region-specific primary cultures . Additionally, this compound induces a significant increase in H2B acetylation in primary neuronal cell cultures .
Action Environment
Given that this compound is brain-penetrant , it can be inferred that the brain environment could potentially influence its action and efficacy.
Biochemical Analysis
Biochemical Properties
BRD-6929 plays a significant role in biochemical reactions, particularly in the inhibition of HDAC1 and HDAC2 . It interacts with these enzymes, inhibiting them with IC50s of 1 and 8 nM, respectively . The nature of these interactions involves high-affinity binding, with Ki values of 0.2 and 1.5 nM for HDAC1 and HDAC2, respectively .
Cellular Effects
This compound has notable effects on various types of cells and cellular processes. It influences cell function by modulating chromatin and gene expression in the brain . In primary neuronal cell cultures, this compound induces a significant increase in H2B acetylation .
Molecular Mechanism
The molecular mechanism of this compound involves its binding interactions with biomolecules, specifically HDAC1 and HDAC2 . It exerts its effects at the molecular level through the inhibition of these enzymes, leading to alterations in gene expression .
Temporal Effects in Laboratory Settings
In laboratory settings, the effects of this compound change over time. The compound exhibits slow-on/slow-off binding kinetics to HDAC1 and HDAC2, with half-lives (T1/2) of 40 and 80 hours, respectively .
Dosage Effects in Animal Models
The effects of this compound vary with different dosages in animal models. For instance, following chronic treatment, this compound at a dosage of 45 mg/kg significantly reduced hyperlocomotion by 36% .
Metabolic Pathways
The metabolic pathways that this compound is involved in are primarily related to the deacetylation of histone and non-histone proteins . It interacts with enzymes such as HDAC1 and HDAC2, influencing the epigenetic regulation of gene expression .
Preparation Methods
Synthetic Routes and Reaction Conditions
The synthesis of JQ12 involves several steps, starting from commercially available starting materials. The key steps typically include:
Formation of the Core Structure: The core structure of JQ12 is synthesized through a series of condensation and cyclization reactions.
Functional Group Modifications: Various functional groups are introduced to the core structure through substitution reactions.
Purification: The final product is purified using techniques such as recrystallization or chromatography to obtain JQ12 in its pure form.
Industrial Production Methods
Industrial production of JQ12 follows similar synthetic routes but on a larger scale. The process involves optimizing reaction conditions to maximize yield and purity while minimizing costs and environmental impact. Techniques such as continuous flow chemistry and automated synthesis may be employed to enhance efficiency and scalability .
Chemical Reactions Analysis
Types of Reactions
JQ12 undergoes several types of chemical reactions, including:
Oxidation: JQ12 can be oxidized under specific conditions to form oxidized derivatives.
Reduction: Reduction reactions can be used to modify certain functional groups within the JQ12 molecule.
Substitution: JQ12 can undergo substitution reactions where specific atoms or groups are replaced with other atoms or groups.
Common Reagents and Conditions
Oxidation: Common oxidizing agents include potassium permanganate and hydrogen peroxide.
Reduction: Reducing agents such as sodium borohydride and lithium aluminum hydride are used.
Substitution: Reagents like halogens, alkylating agents, and nucleophiles are employed in substitution reactions.
Major Products Formed
The major products formed from these reactions depend on the specific reagents and conditions used. For example, oxidation of JQ12 may yield hydroxylated or ketone derivatives, while reduction may produce alcohols or amines .
Scientific Research Applications
JQ12 has a wide range of scientific research applications, including:
Chemistry: JQ12 is used as a chemical tool to study the role of histone deacetylases in gene regulation and chromatin remodeling.
Biology: In biological research, JQ12 is employed to investigate the effects of histone deacetylase inhibition on cellular processes such as differentiation, proliferation, and apoptosis.
Medicine: JQ12 has shown potential as a therapeutic agent in the treatment of various cancers, including leukemia, lymphoma, and solid tumors. It is also being explored for its potential in treating neurodegenerative diseases and inflammatory conditions.
Comparison with Similar Compounds
Similar Compounds
MS-275: Another selective inhibitor of histone deacetylase 1 and histone deacetylase 2, with similar applications in cancer research.
SAHA (Vorinostat): A pan-histone deacetylase inhibitor used in the treatment of cutaneous T-cell lymphoma.
Romidepsin: A selective inhibitor of histone deacetylase 1 and histone deacetylase 2, used in the treatment of peripheral T-cell lymphoma.
Uniqueness of JQ12
JQ12 is unique in its high selectivity for histone deacetylase 1 and histone deacetylase 2, which allows for more targeted modulation of gene expression with potentially fewer side effects compared to pan-histone deacetylase inhibitors. This selectivity makes JQ12 a valuable tool in both basic research and therapeutic development .
Properties
IUPAC Name |
4-acetamido-N-(2-amino-5-thiophen-2-ylphenyl)benzamide | |
---|---|---|
Source | PubChem | |
URL | https://pubchem.ncbi.nlm.nih.gov | |
Description | Data deposited in or computed by PubChem | |
InChI |
InChI=1S/C19H17N3O2S/c1-12(23)21-15-7-4-13(5-8-15)19(24)22-17-11-14(6-9-16(17)20)18-3-2-10-25-18/h2-11H,20H2,1H3,(H,21,23)(H,22,24) | |
Source | PubChem | |
URL | https://pubchem.ncbi.nlm.nih.gov | |
Description | Data deposited in or computed by PubChem | |
InChI Key |
ABZSPJVXTTUFAA-UHFFFAOYSA-N | |
Source | PubChem | |
URL | https://pubchem.ncbi.nlm.nih.gov | |
Description | Data deposited in or computed by PubChem | |
Canonical SMILES |
CC(=O)NC1=CC=C(C=C1)C(=O)NC2=C(C=CC(=C2)C3=CC=CS3)N | |
Source | PubChem | |
URL | https://pubchem.ncbi.nlm.nih.gov | |
Description | Data deposited in or computed by PubChem | |
Molecular Formula |
C19H17N3O2S | |
Source | PubChem | |
URL | https://pubchem.ncbi.nlm.nih.gov | |
Description | Data deposited in or computed by PubChem | |
Molecular Weight |
351.4 g/mol | |
Source | PubChem | |
URL | https://pubchem.ncbi.nlm.nih.gov | |
Description | Data deposited in or computed by PubChem | |
Retrosynthesis Analysis
AI-Powered Synthesis Planning: Our tool employs the Template_relevance Pistachio, Template_relevance Bkms_metabolic, Template_relevance Pistachio_ringbreaker, Template_relevance Reaxys, Template_relevance Reaxys_biocatalysis model, leveraging a vast database of chemical reactions to predict feasible synthetic routes.
One-Step Synthesis Focus: Specifically designed for one-step synthesis, it provides concise and direct routes for your target compounds, streamlining the synthesis process.
Accurate Predictions: Utilizing the extensive PISTACHIO, BKMS_METABOLIC, PISTACHIO_RINGBREAKER, REAXYS, REAXYS_BIOCATALYSIS database, our tool offers high-accuracy predictions, reflecting the latest in chemical research and data.
Strategy Settings
Precursor scoring | Relevance Heuristic |
---|---|
Min. plausibility | 0.01 |
Model | Template_relevance |
Template Set | Pistachio/Bkms_metabolic/Pistachio_ringbreaker/Reaxys/Reaxys_biocatalysis |
Top-N result to add to graph | 6 |
Feasible Synthetic Routes
Q1: The research paper mentions that Merck60, in combination with Tazemetostat, effectively suppressed cell growth in G401 kidney cancer cells. What is the scientific basis for combining a HDAC1/2 inhibitor with an EZH2 inhibitor in the context of Wilms tumor?
A1: The rationale for combining Merck60 (HDAC1/2 inhibitor) and Tazemetostat (EZH2 inhibitor) stems from the study's findings that both HDAC1/2 and EZH2 are overactive in Wilms tumor cells []. HDAC1/2, by upregulating SIX1/2, and EZH2, by promoting nephron progenitor cell proliferation, contribute to the uncontrolled growth characteristic of this cancer []. By inhibiting both these pathways simultaneously, the researchers aimed to achieve a synergistic effect, effectively disrupting the tumor cells' ability to proliferate. This approach aligns with the growing body of evidence suggesting that combined inhibition of HDACs and EZH2 can be a potent strategy for treating various cancers [].
Disclaimer and Information on In-Vitro Research Products
Please be aware that all articles and product information presented on BenchChem are intended solely for informational purposes. The products available for purchase on BenchChem are specifically designed for in-vitro studies, which are conducted outside of living organisms. In-vitro studies, derived from the Latin term "in glass," involve experiments performed in controlled laboratory settings using cells or tissues. It is important to note that these products are not categorized as medicines or drugs, and they have not received approval from the FDA for the prevention, treatment, or cure of any medical condition, ailment, or disease. We must emphasize that any form of bodily introduction of these products into humans or animals is strictly prohibited by law. It is essential to adhere to these guidelines to ensure compliance with legal and ethical standards in research and experimentation.