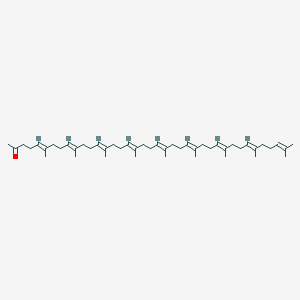
Solanesyl Acetone
Overview
Description
The compound (5E,9E,13E,17E,21E,25E,29E,33E)-6,10,14,18,22,26,30,34,38-nonamethylnonatriaconta-5,9,13,17,21,25,29,33,37-nonaen-2-one is a complex organic molecule with a long chain of carbon atoms and multiple double bonds. Solanesyl Acetone . This compound is notable for its role as an intermediate in the synthesis of Isodecaprenol, a side chain of coenzyme Q10.
Preparation Methods
Synthetic Routes and Reaction Conditions
The synthesis of (5E,9E,13E,17E,21E,25E,29E,33E)-6,10,14,18,22,26,30,34,38-nonamethylnonatriaconta-5,9,13,17,21,25,29,33,37-nonaen-2-one involves multiple steps, typically starting from simpler organic molecules. The process often includes the use of reagents such as DCM (Dichloromethane) and Methanol . The exact synthetic route can vary, but it generally involves the formation of multiple double bonds and the addition of methyl groups at specific positions along the carbon chain.
Industrial Production Methods
In an industrial setting, the production of this compound may involve large-scale chemical reactors and precise control of reaction conditions to ensure high yield and purity. The use of catalysts and optimized reaction times and temperatures are crucial for efficient production.
Chemical Reactions Analysis
Types of Reactions
(5E,9E,13E,17E,21E,25E,29E,33E)-6,10,14,18,22,26,30,34,38-nonamethylnonatriaconta-5,9,13,17,21,25,29,33,37-nonaen-2-one: can undergo various chemical reactions, including:
Oxidation: This reaction can introduce oxygen-containing functional groups into the molecule.
Reduction: This reaction can remove double bonds or reduce other functional groups.
Substitution: This reaction can replace one functional group with another.
Common Reagents and Conditions
Common reagents used in these reactions include oxidizing agents like potassium permanganate and reducing agents like lithium aluminum hydride. Reaction conditions such as temperature, solvent, and pH are carefully controlled to achieve the desired products.
Major Products Formed
The major products formed from these reactions depend on the specific conditions and reagents used. For example, oxidation might yield ketones or alcohols, while reduction could produce alkanes or other reduced forms of the molecule.
Scientific Research Applications
Antioxidant and Anti-inflammatory Properties
Solanesyl acetone exhibits significant antioxidant and anti-inflammatory activities. Research indicates that compounds derived from solanesol can alleviate oxidative stress and reduce pro-inflammatory cytokines, making them candidates for therapeutic agents in conditions like periodontitis .
Drug Delivery Systems
This compound has been utilized in the development of drug delivery systems, particularly for hydrophobic drugs. Micelles formed from solanesol derivatives have shown promise in enhancing the bioavailability of poorly soluble drugs such as coenzyme Q10 and doxorubicin. These micelles improve pharmacokinetic properties and target tumor sites effectively .
Anticancer Applications
Recent studies have highlighted the potential of this compound in anticancer therapies. Derivatives of solanesol have been synthesized to enhance the efficacy of traditional anticancer drugs while minimizing toxicity. For instance, novel diacid solanesyl 5-fluorouracil ester derivatives have demonstrated promising antitumor activity with reduced side effects .
Ubiquinone Biosynthesis
This compound plays a critical role in the biosynthesis of ubiquinones, essential compounds involved in mitochondrial electron transport. The enzyme solanesyl diphosphate synthase (TbSPPS) utilizes solanesyl compounds to produce ubiquinone-9, which is vital for cellular respiration in organisms such as Trypanosoma brucei, the causative agent of sleeping sickness .
Genetic Engineering and Synthetic Biology
The versatility of this compound extends to genetic engineering applications where it serves as a scaffold for constructing molecular probes and ligands. These constructs can be employed in competitive binding assays to study receptor interactions and potentially lead to the development of new therapeutic agents .
Summary of Bioactivities
Drug Delivery Efficacy
Drug | Delivery System | Bioavailability Improvement | References |
---|---|---|---|
Coenzyme Q10 | Solanesol-derived micelles | 300% increase | |
Doxorubicin | Amphiphilic redox-responsive micelles | Enhanced targeting capability |
Case Study: Solanesyl Micelles for Coenzyme Q10
A study demonstrated that solanesyl poly(ethylene glycol) succinate (SPGS) micelles significantly improved the oral bioavailability of coenzyme Q10 in rat models compared to coarse suspensions, indicating the potential for clinical applications in enhancing nutrient absorption .
Case Study: Anticancer Derivatives
Research into diacid solanesyl 5-fluorouracil ester derivatives revealed that these compounds not only retained high antitumor activity but also exhibited low toxicity profiles, making them suitable candidates for further development in cancer therapy .
Mechanism of Action
The mechanism of action of (5E,9E,13E,17E,21E,25E,29E,33E)-6,10,14,18,22,26,30,34,38-nonamethylnonatriaconta-5,9,13,17,21,25,29,33,37-nonaen-2-one involves its interaction with molecular targets in biochemical pathways. As an intermediate in the synthesis of Isodecaprenol, it contributes to the formation of coenzyme Q10, which is essential for cellular energy production and antioxidant protection.
Comparison with Similar Compounds
Similar compounds to (5E,9E,13E,17E,21E,25E,29E,33E)-6,10,14,18,22,26,30,34,38-nonamethylnonatriaconta-5,9,13,17,21,25,29,33,37-nonaen-2-one include:
- (5E,9E,13E,17E,21E,25E,29E,33E)-2,6,10,14,18,22,26,30,34,38-Decamethyl-1,5,9,13,17,21,25,29,33,37-nonatriacontadecaene
- (all-E)-6,10,14,18,22,26,30,34,38-Nonamethyl-5,9,13,17,21,25,29,33,37-nonatriacontanonaen-2-one
These compounds share similar structural features but differ in specific functional groups or the arrangement of double bonds, which can affect their chemical properties and applications.
Biological Activity
Solanesyl acetone, a derivative of solanesol, is a compound of interest due to its potential biological activities. This article delves into the various aspects of this compound, including its antioxidant properties, neuroprotective effects, and applications in drug development.
Overview of this compound
This compound is an isoprenoid compound that has garnered attention for its role in cellular processes and potential therapeutic applications. It is synthesized from solanesol, which is known for its various bioactivities. Understanding the biological activity of this compound can provide insights into its utility in medicine and health.
Antioxidant Activity
One of the primary biological activities associated with this compound is its antioxidant capacity . Research indicates that solanesyl exhibits strong free radical scavenging abilities, comparable to well-known antioxidants like Trolox. The presence of multiple non-conjugated double bonds in its structure contributes to this property, allowing it to effectively neutralize harmful reactive oxygen species (ROS) such as superoxide anions and hydroxyl radicals .
Table 1: Antioxidant Activity Comparison
Neuroprotective Effects
This compound has been studied for its neuroprotective effects , particularly in models of oxidative stress and neurodegeneration. In experimental studies, solanesyl treatment has been shown to alleviate oxidative stress and reduce pro-inflammatory cytokine levels in animal models of periodontitis . Additionally, it has been linked to improved cognitive function and motor performance in models resembling Huntington's disease symptoms, suggesting a protective role against neurodegenerative conditions .
The mechanisms underlying the biological activities of this compound involve several pathways:
- Activation of Nrf2 Pathway : Solanesyl has been shown to activate the Nrf2 pathway, which plays a critical role in cellular defense against oxidative stress. This activation leads to increased expression of heme oxygenase-1 (HO-1), contributing to its anti-inflammatory effects .
- Interaction with Proteins : Studies indicate that solanesyl can bind to proteins such as bovine serum albumin through hydrophobic interactions, which may enhance its bioavailability and therapeutic efficacy .
Applications in Drug Development
The potential applications of this compound extend into drug formulation and cancer therapy:
- Drug Carrier Systems : Solanesyl derivatives have been explored as components in redox-responsive drug delivery systems. These systems can enhance the bioavailability and therapeutic index of chemotherapeutic agents like doxorubicin .
- Anticancer Properties : Research has indicated that solanesyl compounds can modify traditional anticancer drugs to improve their efficacy while reducing toxicity. For instance, synthesized derivatives have shown promising antitumor activity with low toxicity profiles .
Case Study 1: Neuroprotection in Huntington's Disease Models
In a study examining the effects of solanesyl on Huntington's disease-like symptoms induced by 3-nitropropionic acid in rats, researchers found significant improvements in motor performance and cognitive tasks following solanesyl treatment. Histopathological analyses revealed reduced inflammatory markers and oxidative damage in brain tissues, supporting the compound's neuroprotective potential .
Case Study 2: Antioxidant Efficacy in Periodontitis
A recent study demonstrated that intragastric administration of solanesyl significantly reduced systemic oxidative stress markers and pro-inflammatory cytokines in rats with ligature-induced periodontitis. These findings suggest that solanesyl could be beneficial for managing inflammatory conditions related to periodontal disease .
Properties
IUPAC Name |
(5E,9E,13E,17E,21E,25E,29E,33E)-6,10,14,18,22,26,30,34,38-nonamethylnonatriaconta-5,9,13,17,21,25,29,33,37-nonaen-2-one | |
---|---|---|
Source | PubChem | |
URL | https://pubchem.ncbi.nlm.nih.gov | |
Description | Data deposited in or computed by PubChem | |
InChI |
InChI=1S/C48H78O/c1-39(2)21-12-22-40(3)23-13-24-41(4)25-14-26-42(5)27-15-28-43(6)29-16-30-44(7)31-17-32-45(8)33-18-34-46(9)35-19-36-47(10)37-20-38-48(11)49/h21,23,25,27,29,31,33,35,37H,12-20,22,24,26,28,30,32,34,36,38H2,1-11H3/b40-23+,41-25+,42-27+,43-29+,44-31+,45-33+,46-35+,47-37+ | |
Source | PubChem | |
URL | https://pubchem.ncbi.nlm.nih.gov | |
Description | Data deposited in or computed by PubChem | |
InChI Key |
RBIVRAJPGJDFMS-NSCWJZNLSA-N | |
Source | PubChem | |
URL | https://pubchem.ncbi.nlm.nih.gov | |
Description | Data deposited in or computed by PubChem | |
Canonical SMILES |
CC(=CCCC(=CCCC(=CCCC(=CCCC(=CCCC(=CCCC(=CCCC(=CCCC(=CCCC(=O)C)C)C)C)C)C)C)C)C)C | |
Source | PubChem | |
URL | https://pubchem.ncbi.nlm.nih.gov | |
Description | Data deposited in or computed by PubChem | |
Isomeric SMILES |
CC(=CCC/C(=C/CC/C(=C/CC/C(=C/CC/C(=C/CC/C(=C/CC/C(=C/CC/C(=C/CC/C(=C/CCC(=O)C)/C)/C)/C)/C)/C)/C)/C)/C)C | |
Source | PubChem | |
URL | https://pubchem.ncbi.nlm.nih.gov | |
Description | Data deposited in or computed by PubChem | |
Molecular Formula |
C48H78O | |
Source | PubChem | |
URL | https://pubchem.ncbi.nlm.nih.gov | |
Description | Data deposited in or computed by PubChem | |
DSSTOX Substance ID |
DTXSID301316233 | |
Record name | Solanesyl acetone | |
Source | EPA DSSTox | |
URL | https://comptox.epa.gov/dashboard/DTXSID301316233 | |
Description | DSSTox provides a high quality public chemistry resource for supporting improved predictive toxicology. | |
Molecular Weight |
671.1 g/mol | |
Source | PubChem | |
URL | https://pubchem.ncbi.nlm.nih.gov | |
Description | Data deposited in or computed by PubChem | |
CAS No. |
65717-26-2 | |
Record name | Solanesyl acetone | |
Source | CAS Common Chemistry | |
URL | https://commonchemistry.cas.org/detail?cas_rn=65717-26-2 | |
Description | CAS Common Chemistry is an open community resource for accessing chemical information. Nearly 500,000 chemical substances from CAS REGISTRY cover areas of community interest, including common and frequently regulated chemicals, and those relevant to high school and undergraduate chemistry classes. This chemical information, curated by our expert scientists, is provided in alignment with our mission as a division of the American Chemical Society. | |
Explanation | The data from CAS Common Chemistry is provided under a CC-BY-NC 4.0 license, unless otherwise stated. | |
Record name | Solanesyl acetone | |
Source | EPA DSSTox | |
URL | https://comptox.epa.gov/dashboard/DTXSID301316233 | |
Description | DSSTox provides a high quality public chemistry resource for supporting improved predictive toxicology. | |
Record name | 5,9,13,17,21,25,29,33,37-Nonatriacontanonaen-2-one, 6,10,14,18,22,26,30,34,38-nonamethyl-, (5E,9E,13E,17E,21E,25E,29E,33E) | |
Source | European Chemicals Agency (ECHA) | |
URL | https://echa.europa.eu/information-on-chemicals | |
Description | The European Chemicals Agency (ECHA) is an agency of the European Union which is the driving force among regulatory authorities in implementing the EU's groundbreaking chemicals legislation for the benefit of human health and the environment as well as for innovation and competitiveness. | |
Explanation | Use of the information, documents and data from the ECHA website is subject to the terms and conditions of this Legal Notice, and subject to other binding limitations provided for under applicable law, the information, documents and data made available on the ECHA website may be reproduced, distributed and/or used, totally or in part, for non-commercial purposes provided that ECHA is acknowledged as the source: "Source: European Chemicals Agency, http://echa.europa.eu/". Such acknowledgement must be included in each copy of the material. ECHA permits and encourages organisations and individuals to create links to the ECHA website under the following cumulative conditions: Links can only be made to webpages that provide a link to the Legal Notice page. | |
Disclaimer and Information on In-Vitro Research Products
Please be aware that all articles and product information presented on BenchChem are intended solely for informational purposes. The products available for purchase on BenchChem are specifically designed for in-vitro studies, which are conducted outside of living organisms. In-vitro studies, derived from the Latin term "in glass," involve experiments performed in controlled laboratory settings using cells or tissues. It is important to note that these products are not categorized as medicines or drugs, and they have not received approval from the FDA for the prevention, treatment, or cure of any medical condition, ailment, or disease. We must emphasize that any form of bodily introduction of these products into humans or animals is strictly prohibited by law. It is essential to adhere to these guidelines to ensure compliance with legal and ethical standards in research and experimentation.