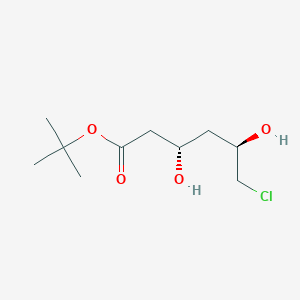
tert-Butyl (3S,4R)-6-Chloro-3,5-dihydroxyhexanoate
- Click on QUICK INQUIRY to receive a quote from our team of experts.
- With the quality product at a COMPETITIVE price, you can focus more on your research.
Overview
Description
(3S,5R)-3,5-Dihydroxy-6-chlorohexanoic acid tert-butyl ester is a chiral compound with significant potential in various scientific fields. This compound is characterized by its two hydroxyl groups, a chlorine atom, and a tert-butyl ester group, making it a versatile molecule for chemical synthesis and research applications.
Preparation Methods
Synthetic Routes and Reaction Conditions
The synthesis of (3S,5R)-3,5-Dihydroxy-6-chlorohexanoic acid tert-butyl ester typically involves the following steps:
Starting Materials: The synthesis begins with the selection of appropriate starting materials, such as 6-chlorohexanoic acid and tert-butyl alcohol.
Esterification: The esterification reaction is carried out by reacting 6-chlorohexanoic acid with tert-butyl alcohol in the presence of an acid catalyst, such as sulfuric acid or p-toluenesulfonic acid, to form the tert-butyl ester.
Hydroxylation: The hydroxylation of the intermediate compound is achieved using a suitable oxidizing agent, such as osmium tetroxide or potassium permanganate, to introduce the hydroxyl groups at the 3 and 5 positions.
Chirality Induction: The chiral centers at positions 3 and 5 are introduced using chiral catalysts or chiral auxiliaries to ensure the desired stereochemistry.
Industrial Production Methods
Industrial production of (3S,5R)-3,5-Dihydroxy-6-chlorohexanoic acid tert-butyl ester involves large-scale synthesis using optimized reaction conditions to maximize yield and purity. The process may include continuous flow reactors, automated systems, and advanced purification techniques such as chromatography and crystallization.
Chemical Reactions Analysis
Types of Reactions
(3S,5R)-3,5-Dihydroxy-6-chlorohexanoic acid tert-butyl ester undergoes various chemical reactions, including:
Oxidation: The hydroxyl groups can be oxidized to form ketones or carboxylic acids using oxidizing agents like chromium trioxide or potassium permanganate.
Reduction: The ester group can be reduced to an alcohol using reducing agents such as lithium aluminum hydride or sodium borohydride.
Substitution: The chlorine atom can be substituted with other functional groups through nucleophilic substitution reactions using reagents like sodium azide or potassium cyanide.
Common Reagents and Conditions
Oxidation: Chromium trioxide in acetic acid, potassium permanganate in water.
Reduction: Lithium aluminum hydride in ether, sodium borohydride in methanol.
Substitution: Sodium azide in DMF, potassium cyanide in ethanol.
Major Products Formed
Oxidation: Formation of 3,5-dioxo-6-chlorohexanoic acid tert-butyl ester.
Reduction: Formation of 3,5-dihydroxy-6-chlorohexanol.
Substitution: Formation of 3,5-dihydroxy-6-azidohexanoic acid tert-butyl ester or 3,5-dihydroxy-6-cyanohexanoic acid tert-butyl ester.
Scientific Research Applications
(3S,5R)-3,5-Dihydroxy-6-chlorohexanoic acid tert-butyl ester has diverse applications in scientific research:
Chemistry: Used as a building block for the synthesis of complex organic molecules and as a chiral auxiliary in asymmetric synthesis.
Biology: Investigated for its potential as a biochemical probe to study enzyme mechanisms and metabolic pathways.
Medicine: Explored for its potential as a drug intermediate in the synthesis of pharmaceuticals with chiral centers.
Industry: Utilized in the production of specialty chemicals and materials with specific stereochemistry requirements.
Mechanism of Action
The mechanism of action of (3S,5R)-3,5-Dihydroxy-6-chlorohexanoic acid tert-butyl ester involves its interaction with specific molecular targets and pathways. The hydroxyl groups and the chlorine atom play crucial roles in its reactivity and binding affinity. The compound may act as an enzyme inhibitor or activator, modulating biochemical pathways and cellular processes.
Comparison with Similar Compounds
Similar Compounds
(3S,5R)-3,5-Dihydroxyhexanoic acid tert-butyl ester: Lacks the chlorine atom, resulting in different reactivity and applications.
(3S,5R)-3,5-Dihydroxy-6-bromohexanoic acid tert-butyl ester: Contains a bromine atom instead of chlorine, leading to variations in chemical behavior.
(3S,5R)-3,5-Dihydroxy-6-fluorohexanoic acid tert-butyl ester: Contains a fluorine atom, which affects its stability and reactivity.
Uniqueness
(3S,5R)-3,5-Dihydroxy-6-chlorohexanoic acid tert-butyl ester is unique due to the presence of both hydroxyl groups and a chlorine atom, which confer distinct chemical properties and reactivity. Its chiral centers make it valuable for applications requiring specific stereochemistry.
Biological Activity
tert-Butyl (3S,4R)-6-chloro-3,5-dihydroxyhexanoate is a compound of significant interest in medicinal chemistry and biochemistry due to its unique structural features and potential biological activities. This article provides a detailed examination of its biological activity, including mechanisms of action, potential therapeutic applications, and relevant research findings.
Structural Characteristics
The compound this compound is characterized by the presence of both hydroxyl and chlorine functional groups. These groups contribute to its reactivity and biological activity, making it a valuable intermediate in the synthesis of pharmaceuticals such as statins.
The biological activity of this compound is primarily mediated through its interaction with specific enzymes and receptors within biological systems. The hydroxyl groups are likely to participate in hydrogen bonding with enzyme active sites, while the chlorine atom may facilitate nucleophilic substitution reactions.
Key Mechanisms Include:
- Enzyme Inhibition : The compound may inhibit enzymes involved in lipid metabolism, which is critical for its role in statin synthesis.
- Antioxidant Activity : Preliminary studies suggest that compounds with similar structures exhibit antioxidant properties, potentially reducing oxidative stress in cells.
Biological Activity Data
Research has demonstrated various biological activities associated with this compound. Below is a summary of significant findings from recent studies:
Case Studies
- Statin Synthesis : A notable application of this compound is its role as an intermediate in the production of rosuvastatin. Research demonstrated that using this compound in synthetic pathways resulted in high yields and enantioselectivity under mild conditions .
- Antioxidant Effects : A study investigated the antioxidant capacity of derivatives similar to this compound. Results indicated that these compounds effectively reduced oxidative stress markers in cellular models .
Research Applications
The unique properties of this compound make it an important compound for:
- Pharmaceutical Development : Its role as an intermediate in statin synthesis highlights its importance in developing cholesterol-lowering medications.
- Biochemical Research : Investigations into its enzyme interactions can provide insights into metabolic pathways and disease mechanisms.
- Industrial Chemistry : The compound's reactivity allows for potential applications in synthesizing specialty chemicals.
Properties
IUPAC Name |
tert-butyl (3S,5R)-6-chloro-3,5-dihydroxyhexanoate |
Source
|
---|---|---|
Source | PubChem | |
URL | https://pubchem.ncbi.nlm.nih.gov | |
Description | Data deposited in or computed by PubChem | |
InChI |
InChI=1S/C10H19ClO4/c1-10(2,3)15-9(14)5-7(12)4-8(13)6-11/h7-8,12-13H,4-6H2,1-3H3/t7-,8+/m0/s1 |
Source
|
Source | PubChem | |
URL | https://pubchem.ncbi.nlm.nih.gov | |
Description | Data deposited in or computed by PubChem | |
InChI Key |
FIKPWJZUGTVXCO-JGVFFNPUSA-N |
Source
|
Source | PubChem | |
URL | https://pubchem.ncbi.nlm.nih.gov | |
Description | Data deposited in or computed by PubChem | |
Canonical SMILES |
CC(C)(C)OC(=O)CC(CC(CCl)O)O |
Source
|
Source | PubChem | |
URL | https://pubchem.ncbi.nlm.nih.gov | |
Description | Data deposited in or computed by PubChem | |
Isomeric SMILES |
CC(C)(C)OC(=O)C[C@H](C[C@H](CCl)O)O |
Source
|
Source | PubChem | |
URL | https://pubchem.ncbi.nlm.nih.gov | |
Description | Data deposited in or computed by PubChem | |
Molecular Formula |
C10H19ClO4 |
Source
|
Source | PubChem | |
URL | https://pubchem.ncbi.nlm.nih.gov | |
Description | Data deposited in or computed by PubChem | |
Molecular Weight |
238.71 g/mol |
Source
|
Source | PubChem | |
URL | https://pubchem.ncbi.nlm.nih.gov | |
Description | Data deposited in or computed by PubChem | |
Disclaimer and Information on In-Vitro Research Products
Please be aware that all articles and product information presented on BenchChem are intended solely for informational purposes. The products available for purchase on BenchChem are specifically designed for in-vitro studies, which are conducted outside of living organisms. In-vitro studies, derived from the Latin term "in glass," involve experiments performed in controlled laboratory settings using cells or tissues. It is important to note that these products are not categorized as medicines or drugs, and they have not received approval from the FDA for the prevention, treatment, or cure of any medical condition, ailment, or disease. We must emphasize that any form of bodily introduction of these products into humans or animals is strictly prohibited by law. It is essential to adhere to these guidelines to ensure compliance with legal and ethical standards in research and experimentation.