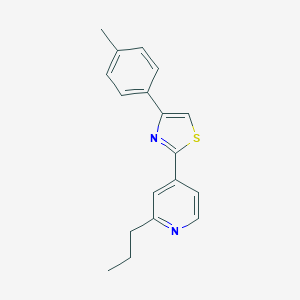
Fatostatin
Overview
Description
Fatostatin A is a synthetic diarylthiazole compound known for its ability to inhibit the activation of sterol regulatory element-binding proteins (SREBPs). SREBPs are transcription factors that regulate lipid metabolism, including the synthesis of cholesterol and fatty acids. By inhibiting SREBPs, this compound A has shown potential in various therapeutic applications, particularly in the treatment of metabolic disorders and cancer .
Mechanism of Action
Target of Action
Fatostatin is a small molecule that primarily targets Sterol Regulatory Element-Binding Proteins (SREBPs), which are key transcription factors controlling lipogenesis and cholesterogenesis . SREBPs regulate genes related to fatty acid and cholesterol biosynthesis . Overexpression of SREBPs has been associated with aggressive pathologic features in human prostate cancer .
Mode of Action
This compound acts by inhibiting the maturation and function of SREBPs . Rather than inhibiting lipogenesis, this compound causes an accumulation of lipids as a response to endoplasmic reticulum stress . In particular, ceramide and dihydroceramide levels increase, contributing to the apoptotic effects of this compound .
Biochemical Pathways
This compound affects several biochemical pathways. It blocks SREBP-regulated metabolic pathways and the Androgen Receptor (AR) signaling network . The main biochemical pathways affected by this compound include purine metabolism, methionine metabolism, glycolysis and gluconeogenesis, tryptophan metabolism, and mitochondrial beta-oxidation of long-chain saturated fatty acids and phospholipid biosynthesis .
Result of Action
This compound has shown significant antitumor activity by suppressing cell proliferation and inducing G2-M cell-cycle arrest and caspase-dependent apoptosis in both androgen-responsive and androgen-insensitive prostate cancer cells . It also reduces in vitro invasion and migration in these cell lines . In vivo, this compound significantly inhibits tumor growth and markedly decreases serum prostate-specific antigen (PSA) levels .
Action Environment
It’s known that the ability of cells to respond to this compound depends on the induction of endoplasmic reticulum stress and subsequent ceramide accumulation . Limiting the production of polyunsaturated fatty acid-containing triacylglycerides (PUFA-TAGs) may enhance the apoptotic effect of this compound, suggesting that these lipids play a protective role and limit this compound response .
Biochemical Analysis
Biochemical Properties
Fatostatin interacts with various enzymes and proteins, primarily through its inhibition of SREBPs . SREBPs are crucial for the production and metabolism of lipids and cholesterol, which are essential for cellular homeostasis and cell proliferation . This compound has been found to inhibit cancer cell proliferation, invasion, migration, and G2/M phase arrest under SREBP-dependent processes .
Cellular Effects
This compound has been shown to have significant effects on various types of cells and cellular processes. It suppresses cell proliferation and anchorage-independent colony formation in both androgen-responsive and androgen-insensitive prostate cancer cells . It also reduces in vitro invasion and migration in these cell lines . Furthermore, this compound has been found to ameliorate inflammation without affecting cell viability .
Molecular Mechanism
This compound exerts its effects at the molecular level primarily through its inhibition of SREBPs . It blocks the activation of SREBPs, thereby inhibiting the transcription of genes related to fatty acid and cholesterol biosynthesis . This leads to a decrease in intracellular cholesterol accumulation and inhibition of XBP1-mediated ER stress .
Temporal Effects in Laboratory Settings
Over time, this compound has been observed to cause an accumulation of lipids in response to endoplasmic reticulum stress rather than inhibition of SREBP activity . This includes an increase in ceramide and dihydroceramide levels, which contribute to the apoptotic effects of this compound .
Dosage Effects in Animal Models
In animal models, this compound has been shown to effectively inhibit the growth of various types of tumors, including B16 melanoma, MC38 colon cancer, and Lewis lung cancer . The effects of this compound vary with different dosages, with high, medium, and low doses (7.5, 15, 30 mg/kg) of this compound administered for sequential drug intervention .
Metabolic Pathways
This compound is involved in various metabolic pathways, primarily those related to lipid metabolism. It reduces SREBPs mediated lipids metabolism in the tumor tissue, especially cholesterol levels . The effects of this compound on the overall metabolic level of the tumor microenvironment mainly focus on tryptophan metabolism, glycolysis and gluconeogenesis metabolism, cysteine and methionine metabolism .
Transport and Distribution
It is known that this compound binds directly to SCAP, blocking its transport from the ER to the Golgi apparatus, thereby inhibiting the activation of SREBPs .
Preparation Methods
Synthetic Routes and Reaction Conditions: Fatostatin A is synthesized through a multi-step process involving the formation of a thiazole ring and subsequent functionalization. The synthesis typically begins with the condensation of a substituted benzaldehyde with thiosemicarbazide to form a thiosemicarbazone intermediate. This intermediate undergoes cyclization with α-bromoacetophenone to yield the thiazole ring. Further functionalization steps, including alkylation and arylation, are performed to obtain the final this compound A compound .
Industrial Production Methods: While detailed industrial production methods for this compound A are not widely documented, the synthesis process can be scaled up using standard organic synthesis techniques. The key steps involve careful control of reaction conditions, purification processes, and quality control measures to ensure high purity and yield of the final product .
Chemical Reactions Analysis
Types of Reactions: Fatostatin A primarily undergoes reactions involving its functional groups, such as the thiazole ring and aromatic substituents. Common reactions include:
Oxidation: this compound A can undergo oxidation reactions, particularly at the thiazole ring, leading to the formation of sulfoxides or sulfones.
Reduction: Reduction reactions can target the aromatic substituents, potentially leading to the formation of reduced derivatives.
Common Reagents and Conditions:
Oxidation: Reagents such as hydrogen peroxide or m-chloroperbenzoic acid can be used under mild conditions.
Reduction: Common reducing agents include sodium borohydride or lithium aluminum hydride.
Substitution: Electrophilic aromatic substitution reactions can be carried out using reagents like bromine or nitric acid.
Major Products Formed: The major products formed from these reactions depend on the specific conditions and reagents used. For example, oxidation of the thiazole ring can yield sulfoxides or sulfones, while substitution reactions can introduce various functional groups onto the aromatic rings .
Scientific Research Applications
Chemistry: Fatostatin A is used as a tool compound to study lipid metabolism and the role of SREBPs in cellular processes.
Comparison with Similar Compounds
Fatostatin A is unique in its ability to inhibit both SREBP-1 and SREBP-2 activation. Similar compounds include:
Betulin: A natural triterpene that inhibits SREBP activation but with a different mechanism of action.
Fibrates: A class of drugs that activate peroxisome proliferator-activated receptors (PPARs) and indirectly affect SREBP activity.
Statins: Cholesterol-lowering drugs that inhibit HMG-CoA reductase, indirectly reducing SREBP activation.
This compound A’s direct inhibition of SREBP activation distinguishes it from these compounds, making it a valuable tool for studying lipid metabolism and a potential therapeutic agent for metabolic disorders and cancer .
Properties
IUPAC Name |
4-(4-methylphenyl)-2-(2-propylpyridin-4-yl)-1,3-thiazole | |
---|---|---|
Source | PubChem | |
URL | https://pubchem.ncbi.nlm.nih.gov | |
Description | Data deposited in or computed by PubChem | |
InChI |
InChI=1S/C18H18N2S/c1-3-4-16-11-15(9-10-19-16)18-20-17(12-21-18)14-7-5-13(2)6-8-14/h5-12H,3-4H2,1-2H3 | |
Source | PubChem | |
URL | https://pubchem.ncbi.nlm.nih.gov | |
Description | Data deposited in or computed by PubChem | |
InChI Key |
ZROSUBKIGBSZCG-UHFFFAOYSA-N | |
Source | PubChem | |
URL | https://pubchem.ncbi.nlm.nih.gov | |
Description | Data deposited in or computed by PubChem | |
Canonical SMILES |
CCCC1=NC=CC(=C1)C2=NC(=CS2)C3=CC=C(C=C3)C | |
Source | PubChem | |
URL | https://pubchem.ncbi.nlm.nih.gov | |
Description | Data deposited in or computed by PubChem | |
Molecular Formula |
C18H18N2S | |
Source | PubChem | |
URL | https://pubchem.ncbi.nlm.nih.gov | |
Description | Data deposited in or computed by PubChem | |
Molecular Weight |
294.4 g/mol | |
Source | PubChem | |
URL | https://pubchem.ncbi.nlm.nih.gov | |
Description | Data deposited in or computed by PubChem | |
CAS No. |
125256-00-0 | |
Record name | Fatostatin | |
Source | European Chemicals Agency (ECHA) | |
URL | https://echa.europa.eu/information-on-chemicals | |
Description | The European Chemicals Agency (ECHA) is an agency of the European Union which is the driving force among regulatory authorities in implementing the EU's groundbreaking chemicals legislation for the benefit of human health and the environment as well as for innovation and competitiveness. | |
Explanation | Use of the information, documents and data from the ECHA website is subject to the terms and conditions of this Legal Notice, and subject to other binding limitations provided for under applicable law, the information, documents and data made available on the ECHA website may be reproduced, distributed and/or used, totally or in part, for non-commercial purposes provided that ECHA is acknowledged as the source: "Source: European Chemicals Agency, http://echa.europa.eu/". Such acknowledgement must be included in each copy of the material. ECHA permits and encourages organisations and individuals to create links to the ECHA website under the following cumulative conditions: Links can only be made to webpages that provide a link to the Legal Notice page. | |
Synthesis routes and methods
Procedure details
Retrosynthesis Analysis
AI-Powered Synthesis Planning: Our tool employs the Template_relevance Pistachio, Template_relevance Bkms_metabolic, Template_relevance Pistachio_ringbreaker, Template_relevance Reaxys, Template_relevance Reaxys_biocatalysis model, leveraging a vast database of chemical reactions to predict feasible synthetic routes.
One-Step Synthesis Focus: Specifically designed for one-step synthesis, it provides concise and direct routes for your target compounds, streamlining the synthesis process.
Accurate Predictions: Utilizing the extensive PISTACHIO, BKMS_METABOLIC, PISTACHIO_RINGBREAKER, REAXYS, REAXYS_BIOCATALYSIS database, our tool offers high-accuracy predictions, reflecting the latest in chemical research and data.
Strategy Settings
Precursor scoring | Relevance Heuristic |
---|---|
Min. plausibility | 0.01 |
Model | Template_relevance |
Template Set | Pistachio/Bkms_metabolic/Pistachio_ringbreaker/Reaxys/Reaxys_biocatalysis |
Top-N result to add to graph | 6 |
Feasible Synthetic Routes
A: Fatostatin is a small molecule that specifically inhibits Sterol Regulatory Element-Binding Protein Cleavage-Activating Protein (SCAP) []. This interaction prevents the endoplasmic reticulum (ER)-to-Golgi transport of SCAP, which is necessary for the activation of Sterol Regulatory Element-Binding Proteins (SREBPs) [, ].
A: Blocking SCAP activation ultimately leads to the inhibition of SREBPs, transcription factors that regulate the expression of genes involved in lipid and cholesterol synthesis and uptake []. This inhibition leads to decreased levels of enzymes involved in these pathways, including:
- Fatty Acid Synthase (FASN): Responsible for the synthesis of fatty acids [, ].
- HMG-CoA Reductase (HMGCR): The rate-limiting enzyme in cholesterol synthesis [].
- Stearoyl-CoA Desaturase 1 (SCD1): Catalyzes the formation of monounsaturated fatty acids [].
A: Interestingly, while this compound potently inhibits cell growth, studies have shown that this effect is not solely dependent on SREBP inhibition and downstream lipogenesis blockade []. This compound was found to inhibit the growth of cells lacking SCAP and also displayed general inhibitory effects on ER-to-Golgi transport [].
A: this compound has been shown to arrest cancer cells in the G2/M phase of the cell cycle [, , ]. This arrest is thought to be linked to its effects on mitotic microtubule spindle assembly and cell division [].
A: this compound has been shown to induce apoptosis in various cancer cell lines [, , , ]. This effect has been linked to:
- Caspase activation: this compound treatment has been shown to increase caspase-3/7 activity and induce the cleavage of caspase-3 and PARP [, ].
- Ceramide production: Lipidomic analysis revealed an increase in ceramide levels in response to this compound, potentially contributing to its pro-apoptotic effects [].
A: While this specific information is not provided in the provided abstracts, the chemical structure of this compound is described as a diarylthiazole derivative [, ]. A more potent derivative, N-(4-(2-(2-propylpyridin-4-yl)thiazol-4-yl)phenyl)methanesulfonamide (FGH10019), has been developed and characterized [].
ANone: The provided research abstracts do not provide specific data on material compatibility or stability of this compound under various conditions.
ANone: this compound is a small molecule inhibitor, not a catalyst. As such, it does not possess catalytic properties or have applications in catalyzing chemical reactions.
A: Yes, in silico screening of chemical databases for compounds with similar effects on SREBF activation as miconazole and clobetasol led to the identification of fenofibrate, a PPARα agonist []. This demonstrates the potential of computational approaches in identifying novel compounds with similar mechanisms of action.
A: Yes, researchers have synthesized and evaluated a series of this compound derivatives to explore structure-activity relationships []. This led to the identification of FGH10019 as a more potent and drug-like molecule with improved aqueous solubility and membrane permeability [].
ANone: The provided research abstracts focus on the preclinical evaluation of this compound and do not contain information regarding SHE regulations or compliance.
ANone: The provided research abstracts primarily focus on the in vitro and in vivo efficacy of this compound. Detailed information regarding its ADME properties is not available in these abstracts.
ANone: this compound has shown promising antitumor activity in a variety of cancer cell lines, including:
- Glioblastoma: T98G, U251, primary-cultured glioblastoma cells (PC38, PC40, PC128), and glioblastoma stem-like cells (SC38, SC40) [, , , ].
- Breast Cancer: MCF-7 and T47D [, ].
- Prostate Cancer: LNCaP and C4-2B [, , , ].
- Pancreatic Cancer: MIA PaCa-2 [, ].
- Endometrial Carcinoma: Specific cell lines not named [].
- Esophageal Carcinoma: OE21 and OE33 [].
ANone: this compound has been shown to:
- Inhibit cell viability and proliferation: Observed in various cancer cell lines [, , , , , , , , , ].
- Suppress anchorage-independent colony formation: Demonstrated in LNCaP and C4-2B prostate cancer cells [, ].
- Reduce migration and invasion: Observed in LNCaP and C4-2B prostate cancer cells and other cell lines [, , , ].
ANone: Yes, this compound has demonstrated antitumor activity in various in vivo models:
- Xenograft models: this compound significantly inhibited tumor growth in mice bearing C4-2B prostate cancer xenografts [, ].
- Syngeneic models: this compound effectively inhibited the growth of B16 melanoma, MC38 colon cancer, and Lewis lung cancer (LLC) tumors in mice [].
ANone: The provided abstracts do not provide information on clinical trials involving this compound.
ANone: The provided abstracts do not offer information regarding specific resistance mechanisms to this compound or potential cross-resistance with other compounds.
A: One study reported that this compound treatment in non-diabetic mice led to hyperfiltration, increased glomerular volume, and increased renal inflammation []. This suggests potential off-target effects and highlights the need for further investigations into its safety profile.
ANone: The provided research abstracts do not provide specific information about strategies for targeted delivery of this compound.
A: Yes, Fatty Acid Synthase (FASN) expression has been identified as a potential predictive biomarker for this compound sensitivity []. High FASN mRNA expression in glioblastoma cells was significantly associated with increased sensitivity to this compound [].
ANone: The provided abstracts mention the use of various analytical techniques to assess the effects of this compound, including:
- qRT-PCR: Used to measure mRNA expression levels of FASN and other genes [, ].
- Western blot: Employed to analyze protein expression levels, including FASN, phosphorylated AKT, and other signaling molecules [, , , ].
- Flow cytometry: Utilized for cell cycle analysis, apoptosis assessment using Annexin V/propidium iodide staining, and other cellular assays [, , , ].
- Immunofluorescence: Used to visualize and analyze cholesterol distribution using filipin and F-actin using phalloidin [].
- Lipidomic analysis: Employed to identify and quantify changes in lipid species following this compound treatment [].
ANone: The provided research abstracts primarily focus on the preclinical evaluation of this compound and do not contain information regarding its environmental impact or degradation.
A: While not explicitly stated, the development of the derivative FGH10019 with improved aqueous solubility suggests that solubility might be a limiting factor for this compound [].
ANone: The provided research abstracts do not offer specific details regarding the validation of analytical methods used in the studies.
ANone: The provided research abstracts focus on the scientific findings and do not include details regarding quality control and assurance procedures.
A: One study reported that this compound treatment in tumor-bearing mice led to a decrease in cholesterol accumulation in the tumor microenvironment, particularly in tumor-infiltrating T lymphocytes []. This resulted in a decrease in the proportion of regulatory T cells (Tregs) and exhausted CD8+ T cells, suggesting a potential role for this compound in promoting anti-tumor immunity [].
ANone: The provided abstracts do not provide specific information regarding potential interactions of this compound with drug transporters.
ANone: The provided research abstracts do not provide specific details regarding the potential of this compound to induce or inhibit drug-metabolizing enzymes.
ANone: The provided research abstracts primarily focus on the in vitro and in vivo efficacy of this compound. They do not contain specific information regarding its biocompatibility or biodegradability.
A: While the abstracts do not directly compare this compound with alternative compounds, other SREBP inhibitors exist, such as PF-429242 and Betulin []. Additionally, several other strategies targeting lipid metabolism in cancer are being explored, including:
- FASN inhibitors: For example, C75 [].
- Statins: HMG-CoA reductase inhibitors, such as atorvastatin [].
ANone: The provided research abstracts do not contain information related to recycling or waste management of this compound.
ANone: The research on this compound highlights the use of various models and techniques relevant to cancer research:
- Cell culture: Use of established and primary cell lines, including cancer stem cell models [, , , , , , , , , ].
- Xenograft and syngeneic mouse models: Preclinical models for evaluating antitumor efficacy [, , ].
- Molecular biology techniques: qRT-PCR, Western blotting, siRNA knockdown experiments for studying gene and protein expression [, , , , , , ].
- Imaging techniques: Confocal microscopy for visualizing cellular processes and in vivo imaging in zebrafish models [, , ].
A: The discovery of this compound as a specific SCAP inhibitor represents a significant milestone in understanding and targeting SREBP-regulated lipid metabolism [].
ANone: Research on this compound highlights the intersection of several scientific disciplines, including:
- Biochemistry: Understanding the mechanism of action of this compound involves studying protein-protein interactions, enzymatic activity, and lipid metabolism [, , , ].
- Cell biology: Investigating the effects of this compound on cell proliferation, cell cycle progression, apoptosis, and cellular signaling pathways [, , , , , , , , ].
- Pharmacology: Evaluating the antitumor efficacy of this compound in vitro and in vivo, exploring structure-activity relationships, and optimizing its pharmacological properties [, , , , ].
- Immunology: Investigating the effects of this compound on the tumor microenvironment and anti-tumor immune responses [].
Disclaimer and Information on In-Vitro Research Products
Please be aware that all articles and product information presented on BenchChem are intended solely for informational purposes. The products available for purchase on BenchChem are specifically designed for in-vitro studies, which are conducted outside of living organisms. In-vitro studies, derived from the Latin term "in glass," involve experiments performed in controlled laboratory settings using cells or tissues. It is important to note that these products are not categorized as medicines or drugs, and they have not received approval from the FDA for the prevention, treatment, or cure of any medical condition, ailment, or disease. We must emphasize that any form of bodily introduction of these products into humans or animals is strictly prohibited by law. It is essential to adhere to these guidelines to ensure compliance with legal and ethical standards in research and experimentation.