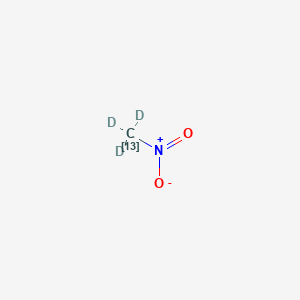
Trideuterio(nitro)(113C)methane
Overview
Description
Trideuterio(nitro)(¹³C)methane (CD₃¹³CNO₂), also known as nitro methane-13C,d3, is a deuterated and carbon-13 isotopically labeled derivative of nitro methane (CH₃NO₂). It has a molecular weight of 65.05120 g/mol and a density of 1.201 g/mL at 25°C . Key physical properties include a boiling point of 101°C, melting point of -29°C, and flash point of 35°C (95°F). The compound is primarily used in spectroscopic studies (e.g., NMR) and tracer experiments due to its isotopic labeling, which minimizes interference in analytical measurements. Its stability requires storage away from strong oxidizers, acids, reducing agents, and copper .
Preparation Methods
Synthetic Routes and Reaction Conditions: Trideuterio(nitro)(113C)methane can be synthesized by reacting nitromethane with deuterium oxide in the presence of bases and phase-transfer catalysts. This reaction forms nitromethane-d3, which is subsequently reduced in an inert solvent to form the desired compound .
Industrial Production Methods: While specific industrial production methods for this compound are not widely documented, the general approach involves the use of deuterium oxide and specialized catalysts to ensure the incorporation of deuterium and carbon-13 isotopes into the nitromethane structure .
Chemical Reactions Analysis
Types of Reactions: Trideuterio(nitro)(113C)methane undergoes various chemical reactions, including:
Oxidation: The nitro group can be oxidized to form nitroalkanes.
Reduction: The nitro group can be reduced to form amines.
Substitution: The nitro group can be substituted with other functional groups under specific conditions.
Common Reagents and Conditions:
Oxidation: Common oxidizing agents include potassium permanganate and chromium trioxide.
Reduction: Common reducing agents include lithium aluminum hydride and hydrogen gas in the presence of a catalyst.
Substitution: Conditions vary depending on the desired substitution but often involve the use of strong bases or acids.
Major Products:
Oxidation: Nitroalkanes.
Reduction: Amines.
Substitution: Various substituted nitromethane derivatives.
Scientific Research Applications
Catalytic Applications
Methane Conversion and Catalysis
One of the most promising applications of trideuterio(nitro)(113C)methane lies in its potential role in catalytic processes for methane conversion. Recent advancements in photocatalytic methods have demonstrated the feasibility of converting methane into methanol under ambient conditions using novel catalysts. For instance, researchers have developed metal-organic frameworks (MOFs) that utilize light to facilitate this transformation, significantly enhancing selectivity and efficiency . The incorporation of isotopically labeled compounds like this compound can aid in tracking reaction pathways and understanding the mechanisms involved in methane activation.
Case Study: Neutron Scattering Techniques
Neutron scattering techniques have been employed to study the interactions between methane and catalysts, revealing insights into bond-weakening interactions crucial for effective catalysis. The use of deuterated methane allows for clearer signals during neutron scattering experiments, improving the understanding of catalytic processes .
Environmental Applications
Methane Mitigation Strategies
this compound may also play a role in environmental applications, particularly in methane mitigation strategies. Research on nitro compounds has shown their efficacy in reducing enteric methane production from livestock. For example, studies involving 3-nitrooxypropanol (3-NOP) have indicated that similar nitro compounds can effectively inhibit methanogenesis in ruminants . By investigating the effects of this compound on microbial populations responsible for methane production, researchers can assess its potential as an additive for reducing greenhouse gas emissions from agriculture.
Table 1: Summary of Nitro Compounds in Methane Mitigation
Compound | Effect on Methane Production | Mechanism of Action |
---|---|---|
3-NOP | Decreases by 30% | Inhibits methanogenic archaea |
This compound | Under investigation | Potentially similar to other nitro compounds |
3-NPA | Reduces by up to 96% | Inhibits production during fermentation |
Biological Research Applications
Tracing Metabolic Pathways
In biological research, this compound can serve as a tracer in metabolic studies. The incorporation of deuterium allows researchers to track the fate of carbon atoms during metabolic processes, providing insights into biochemical pathways involving methane and its derivatives . This application is particularly relevant in studies examining microbial metabolism and energy transfer within ecosystems.
Case Study: Rumen Microbial Interactions
Research has indicated that nitro compounds can alter microbial populations within the rumen, affecting overall fermentation efficiency and methane production. By using this compound as a substrate in rumen fluid cultures, scientists can explore its impact on specific methanogenic archaea and other microbial communities . This approach enables a better understanding of how dietary additives influence methane emissions from livestock.
Mechanism of Action
The mechanism of action of Trideuterio(nitro)(113C)methane involves its interaction with various molecular targets and pathways. The presence of deuterium and carbon-13 isotopes allows for detailed tracking of the compound’s behavior in chemical and biological systems. The nitro group can undergo various reactions, influencing the compound’s reactivity and interactions with other molecules .
Comparison with Similar Compounds
Comparison with Similar Compounds
Structural and Isotopic Variants
Nitro Methane (CH₃NO₂)
- Molecular Formula: CH₃NO₂
- Molecular Weight : 61.04 g/mol
- Density : 1.13 g/mL at 25°C
- Boiling/Melting Points : 101°C (lit.) / -28.5°C
- Applications : Solvent, fuel additive, organic synthesis.
- Key Differences : Lacks isotopic labeling (¹³C and deuterium), resulting in lower molecular weight and density. Reactivity is identical under standard conditions, but kinetic isotope effects may alter reaction rates in deuterated analogs .
Nitro Methane-d₃ (CD₃NO₂)
- Molecular Formula: CD₃NO₂
- Molecular Weight : 64.05 g/mol
- Key Differences: Deuterium substitution reduces vibrational modes in IR spectroscopy and may slow reaction kinetics due to the kinetic isotope effect. No ¹³C labeling limits its utility in carbon-specific tracing.
Nitro Methane-¹³C (CH₃¹³CNO₂)
- Molecular Formula: CH₃¹³CNO₂
- Molecular Weight : 62.04 g/mol
- Key Differences : ¹³C labeling enhances NMR signal resolution but lacks deuterium, making it less suitable for deuterium-based studies.
Physicochemical Properties
Notes:
- Isotopic labeling minimally affects boiling/melting points but significantly alters spectroscopic properties.
- Deuterium increases molecular weight and density due to higher atomic mass compared to protium.
Reactivity and Stability
- Trideuterio(nitro)(¹³C)methane : Decomposes under strong acidic or oxidative conditions, similar to nitro methane. Deuterium substitution may reduce reaction rates in proton-transfer processes .
- Nitro Methane: Known for explosive decomposition under high-pressure or high-temperature conditions with strong bases.
Research Findings and Case Studies
- Spectroscopic Utility : The ¹³C label in Trideuterio(nitro)(¹³C)methane enhances carbon signal resolution in NMR, as seen in studies of isotopically labeled kinase inhibitors (e.g., compound 113c in , though unrelated structurally) .
- Isotopic Fractionation : While discusses ¹³C enrichment in marine ciliates, this highlights the broader relevance of isotopic labeling in environmental and biological tracing .
Biological Activity
Trideuterio(nitro)(113C)methane, a nitro compound, has garnered attention due to its potential biological activities, particularly in the context of methane mitigation and microbial interactions in the rumen. This article reviews the biological activity of this compound, focusing on its effects on methanogenesis and microbial populations, drawing from diverse research findings and case studies.
Chemical Structure and Properties
This compound is a deuterated form of nitromethane, characterized by the presence of three deuterium atoms and a carbon-113 isotope. Its chemical formula is represented as . The structural modifications impart unique properties that influence its biological activity, particularly in ruminal fermentation processes.
Methanogenesis Inhibition
One of the primary biological activities of this compound involves its ability to inhibit methanogenesis in ruminal microbes. Research indicates that nitro compounds can interfere with methane production by affecting the metabolic pathways of methanogenic archaea. For instance, studies have shown that nitro compounds can dock into the active site of Methyl-Coenzyme M reductase, a key enzyme in the methanogenesis pathway, thereby reducing methane output significantly .
Table 1: Effects of Nitro Compounds on Methane Production
Compound | Dose (mM) | Methane Production Reduction (%) |
---|---|---|
This compound | 3 | 29 |
3-Nitropropionic Acid (3NPA) | 6 | 97 |
Ethyl Nitroacetate | 12 | >93 |
Note: Data compiled from various studies illustrating the effectiveness of different nitro compounds in reducing methane production.
Impact on Rumen Microbiome
The introduction of this compound into ruminal cultures has been observed to alter the microbial community structure. Specifically, it tends to reduce the population of hydrogen-producing protozoa, which are integral to methanogenesis. This reduction leads to lower hydrogen availability for methanogenic archaea, further contributing to decreased methane emissions .
Case Study: In Vitro Analysis
In a controlled study assessing the impact of this compound on rumen fermentation, researchers found that at a concentration of 6 mM, there was a significant decrease in total gas production and volatile fatty acids (VFAs), indicating altered fermentation dynamics. The study reported a reduction in methane production by up to 96% compared to untreated controls .
The mechanism by which this compound exerts its effects is believed to involve several biochemical pathways:
- Inhibition of Key Enzymes : As mentioned, it may inhibit enzymes like Methyl-Coenzyme M reductase.
- Alteration of Hydrogen Metabolism : By decreasing hydrogen availability through protozoal inhibition, it shifts metabolic pathways towards more efficient energy utilization routes such as acetogenesis.
- Microbial Adaptation : Over time, microbial populations may adapt to utilize nitro compounds effectively, leading to enhanced degradation rates and altered fermentation profiles.
Safety and Toxicity
While nitro compounds like this compound show promise for methane mitigation, their safety profile must be considered. Studies indicate that moderate doses do not significantly compromise fermentation efficiency or overall microbial health in ruminal environments . However, long-term effects and potential toxicity require further investigation.
Q & A
Basic Research Questions
Q. What synthetic methodologies are recommended for introducing deuterium and ¹³C isotopes into Trideuterio(nitro)(¹³C)methane?
Synthesis of this compound involves isotopic labeling via selective substitution or precursor-based routes. For deuterium incorporation, methods like H/D exchange under acidic or basic conditions using deuterated solvents (e.g., D₂O or CD₃OD) are common. The ¹³C isotope is typically introduced via labeled precursors, such as ¹³C-enriched methyl iodide or methane derivatives. For example, iodomethane-d₃ (trideuterio(iodo)methane) synthesis employs deuterated reagents to replace hydrogen atoms . Analytical validation (e.g., NMR, mass spectrometry) is critical to confirm isotopic purity and positional accuracy .
Q. Which analytical techniques are most effective for characterizing Trideuterio(nitro)(¹³C)methane’s structure and isotopic distribution?
- Nuclear Magnetic Resonance (NMR): ¹H NMR detects residual protons, while ¹³C NMR confirms the position and enrichment of the ¹³C isotope. Deuterium’s quadrupolar moment often broadens signals, requiring specialized ²H NMR or indirect detection methods .
- Mass Spectrometry (MS): High-resolution MS (HRMS) or isotope ratio MS (IRMS) quantifies isotopic abundance and distinguishes between natural abundance and intentional labeling. Fragmentation patterns help verify the nitro group’s presence .
- Isotopic Chromatography: Coupling gas/liquid chromatography with isotopic detectors ensures separation from non-labeled analogs and impurities .
Q. How does isotopic labeling impact the compound’s stability and handling in experimental setups?
Deuterium incorporation can alter kinetic isotope effects (KIEs), affecting reaction rates and thermodynamic stability. For instance, C-D bonds are stronger than C-H bonds, potentially slowing nitro-group reduction or decomposition. Storage in inert, deuterated solvents (e.g., CDCl₃) minimizes unintended H/D exchange. Stability studies under varying pH and temperature are advised to optimize storage conditions .
Advanced Research Questions
Q. How do kinetic isotope effects (KIEs) of Trideuterio(nitro)(¹³C)methane influence its reactivity in catalytic systems compared to non-deuterated analogs?
Deuterium’s KIE (typically 2–7 for C-H vs. C-D bonds) can reduce reaction rates in processes like hydrogenolysis or nitration. For example, in Pd-catalyzed reactions, deuterated methane derivatives may exhibit slower adsorption/desorption kinetics. Computational modeling (DFT) paired with experimental rate comparisons can quantify these effects. Studies on similar compounds, like iodomethane-d₃, show reduced reactivity in SN2 reactions due to deuterium’s mass .
Q. What strategies resolve contradictions in isotopic enrichment data arising from environmental or instrumental variability?
- Calibration with Certified Standards: Use NIST-traceable isotopic standards to normalize MS or IRMS data, correcting for drift or matrix effects .
- Statistical Robustness: Apply wavelet coherence analysis (e.g., WTC) to distinguish true isotopic signals from noise, as demonstrated in ¹³C speleothem studies .
- Cross-Validation: Combine multiple techniques (e.g., NMR for positional confirmation, MS for abundance) to reconcile discrepancies .
Q. How can Trideuterio(nitro)(¹³C)methane be applied in tracing reaction mechanisms or metabolic pathways?
As a stable isotope tracer, it enables real-time monitoring of nitro-group transformations (e.g., reduction to amines) via isotopic mass shifts in MS. In metabolic studies, ¹³C labeling tracks carbon flux in microbial methanogenesis pathways, while deuterium provides insights into hydrogen transfer steps. This dual labeling approach is critical for disentangling complex reaction networks, as seen in studies on methane-oxidizing bacteria .
Q. What experimental design considerations are essential for minimizing isotopic dilution in kinetic studies?
- Reagent Purity: Ensure solvents and catalysts are deuterium-free to prevent H/D exchange.
- Quenching Protocols: Rapidly terminate reactions (e.g., flash-freezing) to halt unintended isotopic scrambling.
- Control Experiments: Compare results with non-labeled analogs to isolate isotope-specific effects .
Q. Data Interpretation and Methodological Challenges
Q. How can researchers differentiate between kinetic and equilibrium isotope effects in studies involving Trideuterio(nitro)(¹³C)methane?
- Kinetic Effects: Measure rate constants (k_H/k_D) under steady-state conditions. For example, slower C-D bond cleavage in nitro-reduction reactions indicates a primary KIE.
- Equilibrium Effects: Use isotopic fractionation experiments (e.g., equilibrium isotopic exchange) to assess thermodynamic preferences. Computational tools like Gaussian or ORCA model bond dissociation energies to predict equilibrium shifts .
Q. What advanced spectral techniques address signal overlap in characterizing deuterated nitro compounds?
- 2D NMR: HSQC or HMBC correlations resolve overlapping ¹H/¹³C signals, particularly in crowded aromatic or nitro regions.
- Dynamic Nuclear Polarization (DNP): Enhances sensitivity for low-abundance isotopes like ¹³C in complex matrices .
Q. How does the isotopic labeling of methane derivatives impact their environmental tracing accuracy in climate studies?
While ¹³C is widely used in paleoclimate reconstructions (e.g., speleothems ), deuterium’s hygroscopicity may introduce biases in atmospheric studies. Dual-labeled compounds require corrections for fractionation during sample preparation (e.g., cryogenic trapping) and analysis.
Properties
IUPAC Name |
trideuterio(nitro)(113C)methane | |
---|---|---|
Source | PubChem | |
URL | https://pubchem.ncbi.nlm.nih.gov | |
Description | Data deposited in or computed by PubChem | |
InChI |
InChI=1S/CH3NO2/c1-2(3)4/h1H3/i1+1D3 | |
Source | PubChem | |
URL | https://pubchem.ncbi.nlm.nih.gov | |
Description | Data deposited in or computed by PubChem | |
InChI Key |
LYGJENNIWJXYER-KQORAOOSSA-N | |
Source | PubChem | |
URL | https://pubchem.ncbi.nlm.nih.gov | |
Description | Data deposited in or computed by PubChem | |
Canonical SMILES |
C[N+](=O)[O-] | |
Source | PubChem | |
URL | https://pubchem.ncbi.nlm.nih.gov | |
Description | Data deposited in or computed by PubChem | |
Isomeric SMILES |
[2H][13C]([2H])([2H])[N+](=O)[O-] | |
Source | PubChem | |
URL | https://pubchem.ncbi.nlm.nih.gov | |
Description | Data deposited in or computed by PubChem | |
Molecular Formula |
CH3NO2 | |
Source | PubChem | |
URL | https://pubchem.ncbi.nlm.nih.gov | |
Description | Data deposited in or computed by PubChem | |
DSSTOX Substance ID |
DTXSID10583511 | |
Record name | Nitro(~13~C,~2~H_3_)methane | |
Source | EPA DSSTox | |
URL | https://comptox.epa.gov/dashboard/DTXSID10583511 | |
Description | DSSTox provides a high quality public chemistry resource for supporting improved predictive toxicology. | |
Molecular Weight |
65.051 g/mol | |
Source | PubChem | |
URL | https://pubchem.ncbi.nlm.nih.gov | |
Description | Data deposited in or computed by PubChem | |
CAS No. |
112898-45-0 | |
Record name | Methane-13C-d3, nitro- | |
Source | CAS Common Chemistry | |
URL | https://commonchemistry.cas.org/detail?cas_rn=112898-45-0 | |
Description | CAS Common Chemistry is an open community resource for accessing chemical information. Nearly 500,000 chemical substances from CAS REGISTRY cover areas of community interest, including common and frequently regulated chemicals, and those relevant to high school and undergraduate chemistry classes. This chemical information, curated by our expert scientists, is provided in alignment with our mission as a division of the American Chemical Society. | |
Explanation | The data from CAS Common Chemistry is provided under a CC-BY-NC 4.0 license, unless otherwise stated. | |
Record name | Nitro(~13~C,~2~H_3_)methane | |
Source | EPA DSSTox | |
URL | https://comptox.epa.gov/dashboard/DTXSID10583511 | |
Description | DSSTox provides a high quality public chemistry resource for supporting improved predictive toxicology. | |
Record name | 112898-45-0 | |
Source | European Chemicals Agency (ECHA) | |
URL | https://echa.europa.eu/information-on-chemicals | |
Description | The European Chemicals Agency (ECHA) is an agency of the European Union which is the driving force among regulatory authorities in implementing the EU's groundbreaking chemicals legislation for the benefit of human health and the environment as well as for innovation and competitiveness. | |
Explanation | Use of the information, documents and data from the ECHA website is subject to the terms and conditions of this Legal Notice, and subject to other binding limitations provided for under applicable law, the information, documents and data made available on the ECHA website may be reproduced, distributed and/or used, totally or in part, for non-commercial purposes provided that ECHA is acknowledged as the source: "Source: European Chemicals Agency, http://echa.europa.eu/". Such acknowledgement must be included in each copy of the material. ECHA permits and encourages organisations and individuals to create links to the ECHA website under the following cumulative conditions: Links can only be made to webpages that provide a link to the Legal Notice page. | |
Disclaimer and Information on In-Vitro Research Products
Please be aware that all articles and product information presented on BenchChem are intended solely for informational purposes. The products available for purchase on BenchChem are specifically designed for in-vitro studies, which are conducted outside of living organisms. In-vitro studies, derived from the Latin term "in glass," involve experiments performed in controlled laboratory settings using cells or tissues. It is important to note that these products are not categorized as medicines or drugs, and they have not received approval from the FDA for the prevention, treatment, or cure of any medical condition, ailment, or disease. We must emphasize that any form of bodily introduction of these products into humans or animals is strictly prohibited by law. It is essential to adhere to these guidelines to ensure compliance with legal and ethical standards in research and experimentation.