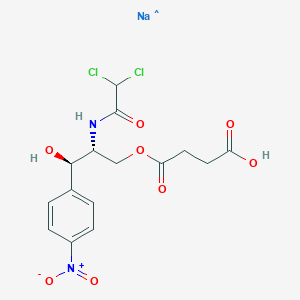
Chloramphenicol sodium succinate
Overview
Description
Chloramphenicol sodium succinate: is a broad-spectrum antibiotic used to treat severe bacterial infections. It is an ester prodrug of chloramphenicol, which means it is converted into the active form, chloramphenicol, in the body. This compound is particularly useful for intravenous administration due to its increased solubility compared to chloramphenicol .
Mechanism of Action
Target of Action
Chloramphenicol sodium succinate is a prodrug of chloramphenicol . The primary target of chloramphenicol is the bacterial ribosome . Specifically, it binds to the residues A2451 and A2452 in the 23S rRNA of the 50S ribosomal subunit of E. coli . This interaction inhibits bacterial protein synthesis, making chloramphenicol a bacteriostatic antibiotic .
Mode of Action
This compound is hydrolyzed into the active chloramphenicol . Chloramphenicol resembles uridine-5’-phosphate . It binds to the bacterial ribosome, blocking the peptidyl transferase step, and preventing the attachment of aminoacyl tRNA to the ribosome . This interaction inhibits bacterial protein synthesis .
Biochemical Pathways
The propane-diol moiety of chloramphenicol can be metabolized through a number of pathways including glucuronidation, sulfate conjugation, phosphorylation, acetylation, and oxidation . The dichloroacetate moiety can be metabolized through hydrolysis of the amide group and dechlorination . The nitro functional group can also be metabolized to an aryl amine and aryl amide metabolite .
Pharmacokinetics
Chloramphenicol succinate acts as a prodrug, being converted to active chloramphenicol while it is circulating in the body . The bioavailability of chloramphenicol after intravenous administration of the succinate ester averages approximately 70%, but the range is quite variable . Incomplete bioavailability is the result of renal excretion of unchanged chloramphenicol succinate prior to it being hydrolyzed to active chloramphenicol . Plasma protein binding of chloramphenicol is approximately 60% in healthy adults . The drug is extensively distributed to many tissues and body fluids, including cerebrospinal fluid and breast milk, and it crosses the placenta . Most of a chloramphenicol dose is metabolized by the liver to inactive products, the chief metabolite being a glucuronide conjugate; only 5 to 15% of chloramphenicol is excreted unchanged in the urine . The elimination half-life is approximately 4 hours .
Result of Action
The result of chloramphenicol’s action is the inhibition of bacterial growth . By binding to the bacterial ribosome and inhibiting protein synthesis, chloramphenicol prevents the bacteria from producing essential proteins, thereby stopping their growth .
Action Environment
The action of this compound can be influenced by environmental factors. For example, the penetration of chloramphenicol in HeLa 3D tumor sphere model was enhanced, which was related to deeply penetrated microemulsion of small size mediated at the tumor site
Biochemical Analysis
Biochemical Properties
Chloramphenicol sodium succinate is hydrolyzed into the active form, chloramphenicol, in the body . Chloramphenicol binds to bacterial ribosomes and prevents translation . It resembles uridine-5’-phosphate and binds to the residues A2451 and A2452 in the 23S rRNA of the 50S ribosomal subunit of E. coli, which prevents translation .
Cellular Effects
This compound, once converted to chloramphenicol, has a broad-spectrum antibiotic effect. It inhibits bacterial protein synthesis by binding to the bacterial ribosome and preventing translation . This action can affect various types of cells and cellular processes, including cell signaling pathways, gene expression, and cellular metabolism.
Molecular Mechanism
The molecular mechanism of action of this compound involves its conversion to Chloramphenicol, which then binds to bacterial ribosomes and inhibits protein synthesis . Chloramphenicol binds to the residues A2451 and A2452 in the 23S rRNA of the 50S ribosomal subunit of E. coli, preventing translation .
Temporal Effects in Laboratory Settings
The effects of this compound change over time in laboratory settings. It is hydrolyzed into the active form, Chloramphenicol, in the body . The bioavailability of Chloramphenicol after intravenous administration of the succinate ester averages approximately 70%, but the range is quite variable .
Dosage Effects in Animal Models
The effects of this compound vary with different dosages in animal models .
Metabolic Pathways
This compound is metabolized (hydrolyzed) by esterases in the body to active Chloramphenicol . Most of a Chloramphenicol dose is metabolized by the liver to inactive products, the chief metabolite being a glucuronide conjugate .
Transport and Distribution
This compound, once converted to Chloramphenicol, is extensively distributed to many tissues and body fluids, including cerebrospinal fluid and breast milk, and it crosses the placenta .
Subcellular Localization
This compound, once converted to Chloramphenicol, is a small molecule that diffuses well into many body tissues including cerebral spinal fluid (CSF) - even in the absence of inflamed meninges, eye, pleural fluid, synovial fluid, ascitic fluid, liver, and kidneys .
Preparation Methods
Synthetic Routes and Reaction Conditions: Chloramphenicol sodium succinate is synthesized by esterifying chloramphenicol with succinic anhydride in the presence of an organic amine catalyst. The reaction involves the formation of an ester bond between the hydroxyl group of chloramphenicol and the carboxyl group of succinic anhydride .
Industrial Production Methods: In industrial settings, this compound is produced by dissolving chloramphenicol in an appropriate solvent and reacting it with succinic anhydride under controlled conditions. The product is then purified and converted into its sodium salt form for pharmaceutical use .
Chemical Reactions Analysis
Types of Reactions: Chloramphenicol sodium succinate undergoes hydrolysis to release the active chloramphenicol. This hydrolysis is catalyzed by esterases in the body .
Common Reagents and Conditions:
Hydrolysis: Water and esterases are the primary reagents involved in the hydrolysis of this compound.
Oxidation and Reduction: this compound does not typically undergo oxidation or reduction reactions under physiological conditions.
Major Products: The primary product of hydrolysis is chloramphenicol, which exerts the antibiotic effect by inhibiting bacterial protein synthesis .
Scientific Research Applications
Chemistry: Chloramphenicol sodium succinate is used in molecular biology for bacterial selection in genetic experiments. It is also employed in the synthesis of various derivatives for research purposes .
Biology: In biological research, this compound is used to study bacterial protein synthesis and the mechanisms of antibiotic resistance .
Medicine: Clinically, this compound is used to treat severe infections caused by susceptible bacteria, including meningitis, typhoid fever, and rickettsial infections .
Industry: In the pharmaceutical industry, this compound is used in the formulation of injectable antibiotics due to its solubility and stability .
Comparison with Similar Compounds
Chloramphenicol: The active form of chloramphenicol sodium succinate, used for oral and topical administration.
Chloramphenicol palmitate: An ester prodrug of chloramphenicol used for oral administration.
Uniqueness: Chloramphenicol sodium succinate is unique due to its solubility and suitability for intravenous administration. Unlike chloramphenicol palmitate, which is used orally, this compound is preferred for severe infections requiring rapid and controlled delivery of the antibiotic .
Biological Activity
Chloramphenicol sodium succinate (CSS) is an ester prodrug of chloramphenicol, a broad-spectrum antibiotic known for its bacteriostatic properties. This article delves into the biological activity of CSS, including its pharmacological mechanisms, clinical applications, and associated toxicities, supported by data tables and case studies.
This compound is metabolized to chloramphenicol, which inhibits bacterial protein synthesis by binding to the 50S ribosomal subunit. Specifically, it targets the A2451 and A2452 residues in the 23S rRNA, preventing peptide bond formation and thus halting bacterial growth . This mechanism makes CSS effective against a variety of Gram-positive and Gram-negative bacteria.
Pharmacokinetics
The pharmacokinetic profile of this compound reveals significant variability among patients. Key parameters include:
Parameter | Value |
---|---|
Volume of Distribution | 0.2 - 3.1 L/kg |
Protein Binding | 57% - 92% |
Half-life | 0.6 - 2.7 hours |
Clearance | 530 - 540 mL/min (normal function) |
Renal Clearance | 222 - 260 mL/min (normal function) |
CSS is primarily eliminated through the urine, with approximately 30% excreted unchanged and about 10% as active chloramphenicol .
Clinical Applications
CSS is indicated for serious bacterial infections where other treatments have failed or are contraindicated. It is particularly effective against:
- Typhoid Fever : CSS has demonstrated efficacy in treating infections caused by Salmonella typhi.
- Meningitis : Due to its ability to penetrate the blood-brain barrier, chloramphenicol is utilized in treating central nervous system infections .
Toxicity and Side Effects
Despite its effectiveness, this compound carries a risk of serious side effects, particularly hematological toxicities:
- Aplastic Anemia : A rare but potentially fatal condition that can develop after treatment.
- Reversible Bone Marrow Suppression : Studies in animal models have shown that CSS can induce reticulocytopenia and anemia, which are reversible upon discontinuation .
Case Studies on Toxicity
- Study on Guinea Pigs : In a controlled study where guinea pigs were administered various doses of CSS, researchers observed dose-related effects on erythrocyte counts and bone marrow activity. At higher doses (2500 mg/kg), significant reductions in reticulocyte counts were noted, indicating bone marrow suppression .
- Human Pharmacokinetic Study : In critically ill patients, CSS kinetics were assessed using high-performance liquid chromatography. Results showed substantial interpatient variability in drug metabolism and clearance rates, emphasizing the need for careful monitoring during treatment .
Resistance Mechanisms
Bacterial resistance to chloramphenicol can occur through several mechanisms:
- Reduced Membrane Permeability : Bacteria may alter their membranes to limit drug entry.
- Ribosomal Mutations : Changes in the ribosomal structure can prevent chloramphenicol binding.
- Chloramphenicol Acetyltransferase Production : Some bacteria produce enzymes that inactivate chloramphenicol by acetylation .
Properties
CAS No. |
982-57-0 |
---|---|
Molecular Formula |
C15H16Cl2N2O8.Na C15H16Cl2N2NaO8 |
Molecular Weight |
446.2 g/mol |
IUPAC Name |
sodium;4-[(2R,3R)-2-[(2,2-dichloroacetyl)amino]-3-hydroxy-3-(4-nitrophenyl)propoxy]-4-oxobutanoate |
InChI |
InChI=1S/C15H16Cl2N2O8.Na/c16-14(17)15(24)18-10(7-27-12(22)6-5-11(20)21)13(23)8-1-3-9(4-2-8)19(25)26;/h1-4,10,13-14,23H,5-7H2,(H,18,24)(H,20,21);/t10-,13-;/m1./s1 |
InChI Key |
OQAWGHRXAVKAIG-HTMVYDOJSA-N |
SMILES |
C1=CC(=CC=C1C(C(COC(=O)CCC(=O)[O-])NC(=O)C(Cl)Cl)O)[N+](=O)[O-].[Na+] |
Isomeric SMILES |
C1=CC(=CC=C1[C@H]([C@@H](COC(=O)CCC(=O)O)NC(=O)C(Cl)Cl)O)[N+](=O)[O-].[Na] |
Canonical SMILES |
C1=CC(=CC=C1C(C(COC(=O)CCC(=O)O)NC(=O)C(Cl)Cl)O)[N+](=O)[O-].[Na] |
Appearance |
White solid |
Key on ui other cas no. |
982-57-0 |
physical_description |
Chloramphenicol sodium succinate is a white powder. (NTP, 1992) |
Pictograms |
Irritant; Health Hazard |
Related CAS |
3544-94-3 (Parent) |
solubility |
greater than or equal to 100 mg/mL at 68.9 °F (NTP, 1992) |
Synonyms |
1-[(2R,3R)-2-[(2,2-Dichloroacetyl)amino]-3-hydroxy-3-(4-nitrophenyl)propyl] Ester Butanedioic Acid Sodium Salt; Mono[(2R,3R)-2-[(dichloroacetyl)amino]-3-hydroxy-3-(4-nitrophenyl)propyl] Ester Butanedioic Acid Monosodium Salt; Betamicetin; Chloramphen |
Origin of Product |
United States |
Retrosynthesis Analysis
AI-Powered Synthesis Planning: Our tool employs the Template_relevance Pistachio, Template_relevance Bkms_metabolic, Template_relevance Pistachio_ringbreaker, Template_relevance Reaxys, Template_relevance Reaxys_biocatalysis model, leveraging a vast database of chemical reactions to predict feasible synthetic routes.
One-Step Synthesis Focus: Specifically designed for one-step synthesis, it provides concise and direct routes for your target compounds, streamlining the synthesis process.
Accurate Predictions: Utilizing the extensive PISTACHIO, BKMS_METABOLIC, PISTACHIO_RINGBREAKER, REAXYS, REAXYS_BIOCATALYSIS database, our tool offers high-accuracy predictions, reflecting the latest in chemical research and data.
Strategy Settings
Precursor scoring | Relevance Heuristic |
---|---|
Min. plausibility | 0.01 |
Model | Template_relevance |
Template Set | Pistachio/Bkms_metabolic/Pistachio_ringbreaker/Reaxys/Reaxys_biocatalysis |
Top-N result to add to graph | 6 |
Feasible Synthetic Routes
A: Chloramphenicol sodium succinate is a prodrug that is hydrolyzed in vivo to Chloramphenicol. [] Chloramphenicol acts by reversibly binding to the 50S subunit of bacterial ribosomes, specifically targeting the peptidyl transferase activity. [] This binding interferes with the elongation process of protein synthesis, effectively preventing bacterial cell growth. []
ANone: While a detailed spectroscopic analysis is not provided in these abstracts, this compound is the sodium succinate ester of Chloramphenicol. This means a succinic acid moiety is attached to the Chloramphenicol molecule, and the carboxyl group of succinic acid is present in its sodium salt form.
A: Research suggests this compound can be loaded onto silicone hydrogel contact lenses, demonstrating potential for sustained release in treating ocular bacterial infections. [] The drug release was found to be directly proportional to the loading concentration. []
ANone: this compound is not known to possess catalytic properties. Its primary application stems from the antibacterial activity of its active metabolite, Chloramphenicol.
ANone: The provided abstracts do not delve into computational chemistry studies or QSAR models for this compound.
A: Information regarding the stability of different this compound formulations is limited in the abstracts. One study mentions that this compound can be hydrolyzed to active Chloramphenicol in ventricular fluid. []
ANone: The provided research abstracts do not specifically address SHE regulations concerning this compound.
A: this compound, when administered intramuscularly, is well absorbed and achieves therapeutic levels in the blood. [] Studies in children showed peak blood levels comparable to those achieved with intravenous administration, suggesting efficient absorption from muscle tissue. []
A: In a study on adult horses, the mean bioavailability of Chloramphenicol base after oral administration was found to be 28%, significantly lower than the bioavailability achieved with intravenous this compound. []
A: Age significantly impacts the pharmacokinetics of Chloramphenicol in foals. Studies show that elimination half-life (t1/2 beta) decreases significantly from 5.29 hours in one-day-old foals to 0.34 hours in 42-day-old foals, indicating faster clearance with increasing age. [] This highlights the importance of age-specific dosing regimens for this drug in horses.
A: Hemodialysis can significantly increase the total body clearance (ClTB) of Chloramphenicol, especially in patients with compromised liver function. [] This increase in clearance during dialysis necessitates careful monitoring of drug levels and potential dose adjustments. []
A: Studies suggest that treatment of bacterial meningitis in children with intramuscular this compound, combined with oral probenecid and chloramphenicol palmitate, can be as effective as intravenous administration of Chloramphenicol. [] This suggests that alternative routes of administration can be considered for this condition.
A: While the provided abstracts do not extensively cover resistance mechanisms, one study investigating Haemophilus species bacteremia in adults highlights a concerning trend of increasing resistance to Chloramphenicol. [] This underscores the importance of monitoring resistance patterns and adapting treatment strategies accordingly.
A: One study explored the possibility of using silicone hydrogel contact lenses as a sustained-release platform for this compound in treating ocular infections. [] This approach holds promise for improving patient compliance and achieving targeted drug delivery to the eye.
ANone: The provided abstracts do not discuss specific biomarkers for predicting efficacy, monitoring treatment response, or identifying adverse effects associated with this compound.
ANone: Several analytical methods are mentioned in the research for measuring Chloramphenicol and its metabolites in various biological matrices:
- High-Performance Liquid Chromatography (HPLC): Utilized for determining Chloramphenicol concentrations in plasma and milk. [, , , ]
- Microbiological Assay: Used to determine plasma chloramphenicol concentrations. []
- Chloramphenicol-ELISA: Employed for monitoring serum chloramphenicol concentrations. []
- Chemical Colorimetric Method: Used to quantify plasma chloramphenicol levels. []
A: Yes, radial-compression liquid chromatography allows for the direct and simultaneous measurement of Chloramphenicol and its monosuccinate ester in minute plasma samples (as small as 10 μL). [] This method offers a rapid and efficient way to analyze both compounds in clinical settings.
ANone: The environmental impact and degradation of this compound are not discussed in the provided abstracts.
ANone: The provided abstracts do not discuss studies specifically addressing the dissolution rate or solubility of this compound in various media.
A: While not extensively detailed, some abstracts mention validation parameters like accuracy, precision, and specificity for the employed analytical methods, emphasizing the importance of reliable and robust analytical techniques. [, ]
ANone: The research papers provided do not delve into the specifics of quality control and assurance measures during the development, manufacturing, or distribution of this compound.
ANone: The abstracts do not discuss the potential of this compound to induce an immune response or any strategies to address immunogenicity concerns.
ANone: The research provided does not provide information on interactions between this compound and drug transporters.
A: Contrary to its effects observed in other species, Chloramphenicol, even at doses causing a significant decrease in the activity of hepatic biotransformation enzymes (aniline hydroxylase and ethylmorphine N-demethylase), did not prolong the duration of pentobarbital or ketamine/xylazine anesthesia in guinea pigs. [] This suggests species-specific differences in drug interactions and highlights the importance of considering species-specific metabolism when extrapolating data.
Disclaimer and Information on In-Vitro Research Products
Please be aware that all articles and product information presented on BenchChem are intended solely for informational purposes. The products available for purchase on BenchChem are specifically designed for in-vitro studies, which are conducted outside of living organisms. In-vitro studies, derived from the Latin term "in glass," involve experiments performed in controlled laboratory settings using cells or tissues. It is important to note that these products are not categorized as medicines or drugs, and they have not received approval from the FDA for the prevention, treatment, or cure of any medical condition, ailment, or disease. We must emphasize that any form of bodily introduction of these products into humans or animals is strictly prohibited by law. It is essential to adhere to these guidelines to ensure compliance with legal and ethical standards in research and experimentation.