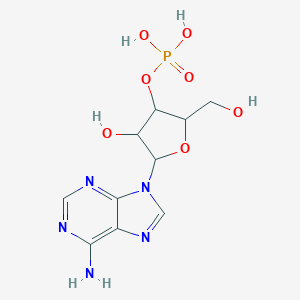
Adenosine 3'-monophosphate hydrate
Overview
Description
Adenosine 3’-monophosphate hydrate is a nucleotide and a metabolite formed via the hydrolysis of 2’,3’-cyclic adenosine monophosphate by metal-dependent phosphodiesterases . It is an ester of phosphoric acid with the nucleoside adenosine, consisting of a phosphate group, the pentose sugar ribose, and the nucleobase adenine . This compound plays a crucial role in various biological processes, including cellular energy transfer and signal transduction.
Mechanism of Action
- Adenosine 3’-monophosphate (3’-AMP) is a metabolite produced from the hydrolysis of 2’,3’-cAMP by a family of metal-dependent phosphodiesterases .
- Its primary targets include adenosine receptors , specifically the A2B receptors . These receptors are found in various cell types, including preglomerular vascular smooth muscle cells and glomerular mesangial cells .
- Upon binding to A2B receptors, it inhibits the proliferation of preglomerular vascular smooth muscle cells and glomerular mesangial cells .
- The exact intracellular pathways triggered by A2B receptor activation are still being explored, but this represents a novel pathway for adenosine-based cell regulation .
- Adenosine is a key regulator in many cellular reactions. It acts as a second messenger in signal transduction mechanisms .
Target of Action
Mode of Action
Biochemical Pathways
Action Environment
Biochemical Analysis
Biochemical Properties
Adenosine 3’-monophosphate hydrate interacts with various enzymes, proteins, and other biomolecules. It is formed via the hydrolysis of 2’,3’-cAMP by metal-dependent phosphodiesterases . This compound reduces the proliferation of certain cells in a concentration-dependent manner .
Cellular Effects
Adenosine 3’-monophosphate hydrate has a profound impact on various types of cells and cellular processes. It influences cell function by modulating cell signaling pathways, gene expression, and cellular metabolism . For instance, it acts as an activator of protein kinase A (PKA), a key regulator of many cellular reactions .
Molecular Mechanism
At the molecular level, Adenosine 3’-monophosphate hydrate exerts its effects through binding interactions with biomolecules and changes in gene expression. It directly activates Epac, a Rap1 guanine-nucleotide exchange factor . It also acts as a second messenger in signal transduction mechanisms .
Temporal Effects in Laboratory Settings
In laboratory settings, the effects of Adenosine 3’-monophosphate hydrate change over time. The hydrates of this compound have been systematically investigated for their crystal structures, humidity sensibility, and transformation behaviors mediated by humidity . The reversible conversions between the hydrates and their corresponding low hydrates or even anhydrates occur along with desorption/uptake of crystal water mediated by humidity .
Metabolic Pathways
Adenosine 3’-monophosphate hydrate is involved in various metabolic pathways. It is a derivative of adenosine triphosphate (ATP), which is a crucial molecule in cellular metabolism . Detailed information about the specific metabolic pathways that this compound is involved in is currently limited.
Preparation Methods
Synthetic Routes and Reaction Conditions
Adenosine 3’-monophosphate hydrate can be synthesized through the hydrolysis of 2’,3’-cyclic adenosine monophosphate using metal-dependent phosphodiesterases . The reaction typically involves the use of a buffer solution and specific metal ions to facilitate the hydrolysis process.
Industrial Production Methods
Industrial production of adenosine 3’-monophosphate hydrate often involves the use of high-performance liquid chromatography (HPLC) for the purification and quantification of the compound . The process includes the extraction of nucleotides from biological samples, followed by separation and purification using HPLC techniques.
Chemical Reactions Analysis
Types of Reactions
Adenosine 3’-monophosphate hydrate undergoes various chemical reactions, including hydrolysis, phosphorylation, and dephosphorylation . These reactions are essential for its role in cellular metabolism and energy transfer.
Common Reagents and Conditions
Phosphorylation: Involves the addition of a phosphate group, often facilitated by enzymes such as kinases.
Dephosphorylation: The removal of a phosphate group, usually catalyzed by phosphatases.
Major Products Formed
Hydrolysis: Produces adenosine and inorganic phosphate.
Phosphorylation: Forms adenosine diphosphate (ADP) or adenosine triphosphate (ATP).
Dephosphorylation: Results in the formation of adenosine and inorganic phosphate.
Scientific Research Applications
Adenosine 3’-monophosphate hydrate has a wide range of applications in scientific research, including:
Comparison with Similar Compounds
Similar Compounds
- Adenosine 2’-monophosphate
- Adenosine 5’-monophosphate
- Cyclic adenosine monophosphate (cAMP)
Uniqueness
Adenosine 3’-monophosphate hydrate is unique due to its specific role in the hydrolysis of 2’,3’-cyclic adenosine monophosphate and its involvement in various biochemical pathways . Unlike other adenosine monophosphates, it has distinct properties and functions that make it valuable in scientific research and industrial applications .
Properties
IUPAC Name |
[(2R,3S,4R,5R)-5-(6-aminopurin-9-yl)-4-hydroxy-2-(hydroxymethyl)oxolan-3-yl] dihydrogen phosphate;hydrate | |
---|---|---|
Source | PubChem | |
URL | https://pubchem.ncbi.nlm.nih.gov | |
Description | Data deposited in or computed by PubChem | |
InChI |
InChI=1S/C10H14N5O7P.H2O/c11-8-5-9(13-2-12-8)15(3-14-5)10-6(17)7(4(1-16)21-10)22-23(18,19)20;/h2-4,6-7,10,16-17H,1H2,(H2,11,12,13)(H2,18,19,20);1H2/t4-,6-,7-,10-;/m1./s1 | |
Source | PubChem | |
URL | https://pubchem.ncbi.nlm.nih.gov | |
Description | Data deposited in or computed by PubChem | |
InChI Key |
HAWIDQLWSQBRQS-MCDZGGTQSA-N | |
Source | PubChem | |
URL | https://pubchem.ncbi.nlm.nih.gov | |
Description | Data deposited in or computed by PubChem | |
Canonical SMILES |
C1=NC(=C2C(=N1)N(C=N2)C3C(C(C(O3)CO)OP(=O)(O)O)O)N.O | |
Source | PubChem | |
URL | https://pubchem.ncbi.nlm.nih.gov | |
Description | Data deposited in or computed by PubChem | |
Isomeric SMILES |
C1=NC(=C2C(=N1)N(C=N2)[C@H]3[C@@H]([C@@H]([C@H](O3)CO)OP(=O)(O)O)O)N.O | |
Source | PubChem | |
URL | https://pubchem.ncbi.nlm.nih.gov | |
Description | Data deposited in or computed by PubChem | |
Molecular Formula |
C10H16N5O8P | |
Source | PubChem | |
URL | https://pubchem.ncbi.nlm.nih.gov | |
Description | Data deposited in or computed by PubChem | |
DSSTOX Substance ID |
DTXSID10871566 | |
Record name | (2R,3S,4R,5R)-5-(6-Amino-9H-purin-9-yl)-4-hydroxy-2-(hydroxymethyl)tetrahydro-3-furanyl dihydrogen phosphate hydrate (1:1) | |
Source | EPA DSSTox | |
URL | https://comptox.epa.gov/dashboard/DTXSID10871566 | |
Description | DSSTox provides a high quality public chemistry resource for supporting improved predictive toxicology. | |
Molecular Weight |
365.24 g/mol | |
Source | PubChem | |
URL | https://pubchem.ncbi.nlm.nih.gov | |
Description | Data deposited in or computed by PubChem | |
CAS No. |
6199-71-9 | |
Record name | (2R,3S,4R,5R)-5-(6-Amino-9H-purin-9-yl)-4-hydroxy-2-(hydroxymethyl)tetrahydro-3-furanyl dihydrogen phosphate hydrate (1:1) | |
Source | EPA DSSTox | |
URL | https://comptox.epa.gov/dashboard/DTXSID10871566 | |
Description | DSSTox provides a high quality public chemistry resource for supporting improved predictive toxicology. | |
Retrosynthesis Analysis
AI-Powered Synthesis Planning: Our tool employs the Template_relevance Pistachio, Template_relevance Bkms_metabolic, Template_relevance Pistachio_ringbreaker, Template_relevance Reaxys, Template_relevance Reaxys_biocatalysis model, leveraging a vast database of chemical reactions to predict feasible synthetic routes.
One-Step Synthesis Focus: Specifically designed for one-step synthesis, it provides concise and direct routes for your target compounds, streamlining the synthesis process.
Accurate Predictions: Utilizing the extensive PISTACHIO, BKMS_METABOLIC, PISTACHIO_RINGBREAKER, REAXYS, REAXYS_BIOCATALYSIS database, our tool offers high-accuracy predictions, reflecting the latest in chemical research and data.
Strategy Settings
Precursor scoring | Relevance Heuristic |
---|---|
Min. plausibility | 0.01 |
Model | Template_relevance |
Template Set | Pistachio/Bkms_metabolic/Pistachio_ringbreaker/Reaxys/Reaxys_biocatalysis |
Top-N result to add to graph | 6 |
Feasible Synthetic Routes
Disclaimer and Information on In-Vitro Research Products
Please be aware that all articles and product information presented on BenchChem are intended solely for informational purposes. The products available for purchase on BenchChem are specifically designed for in-vitro studies, which are conducted outside of living organisms. In-vitro studies, derived from the Latin term "in glass," involve experiments performed in controlled laboratory settings using cells or tissues. It is important to note that these products are not categorized as medicines or drugs, and they have not received approval from the FDA for the prevention, treatment, or cure of any medical condition, ailment, or disease. We must emphasize that any form of bodily introduction of these products into humans or animals is strictly prohibited by law. It is essential to adhere to these guidelines to ensure compliance with legal and ethical standards in research and experimentation.