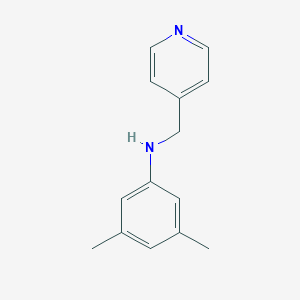
3,5-dimethyl-N-(pyridin-4-ylmethyl)aniline
- Click on QUICK INQUIRY to receive a quote from our team of experts.
- With the quality product at a COMPETITIVE price, you can focus more on your research.
Overview
Description
3,5-Dimethyl-N-(pyridin-4-ylmethyl)aniline is an organic compound with the molecular formula C14H16N2 and a molecular weight of 212.29 g/mol . It is characterized by the presence of a pyridine ring attached to an aniline moiety, with two methyl groups at the 3 and 5 positions of the aniline ring.
Preparation Methods
Synthetic Routes and Reaction Conditions
The synthesis of 3,5-dimethyl-N-(pyridin-4-ylmethyl)aniline typically involves the reaction of 3,5-dimethylaniline with pyridine-4-carboxaldehyde under reductive amination conditions. The reaction is usually carried out in the presence of a reducing agent such as sodium borohydride or hydrogen gas with a suitable catalyst .
Industrial Production Methods
Industrial production of this compound may involve similar synthetic routes but on a larger scale, with optimizations for yield and purity. The use of continuous flow reactors and automated systems can enhance the efficiency and scalability of the production process .
Chemical Reactions Analysis
Types of Reactions
3,5-Dimethyl-N-(pyridin-4-ylmethyl)aniline can undergo various chemical reactions, including:
Oxidation: The compound can be oxidized to form corresponding N-oxides or other oxidized derivatives.
Reduction: Reduction reactions can lead to the formation of reduced amine derivatives.
Substitution: Electrophilic and nucleophilic substitution reactions can occur at the aromatic rings.
Common Reagents and Conditions
Oxidation: Common oxidizing agents include hydrogen peroxide, potassium permanganate, and chromium trioxide.
Reduction: Reducing agents such as sodium borohydride, lithium aluminum hydride, and catalytic hydrogenation are commonly used.
Substitution: Reagents like halogens, alkylating agents, and acylating agents are used under various conditions.
Major Products
The major products formed from these reactions depend on the specific reagents and conditions used. For example, oxidation may yield N-oxides, while substitution reactions can introduce various functional groups onto the aromatic rings .
Scientific Research Applications
3,5-Dimethyl-N-(pyridin-4-ylmethyl)aniline has several applications in scientific research:
Chemistry: It is used as a building block in the synthesis of more complex organic molecules and in the study of reaction mechanisms.
Biology: The compound can be used in the development of bioactive molecules and as a probe in biochemical assays.
Medicine: Research into its potential therapeutic applications, such as in the development of new drugs, is ongoing.
Industry: It is used in the production of dyes, pigments, and other specialty chemicals.
Mechanism of Action
The mechanism of action of 3,5-dimethyl-N-(pyridin-4-ylmethyl)aniline involves its interaction with specific molecular targets, such as enzymes or receptors. The compound can modulate the activity of these targets, leading to various biological effects. The exact pathways and targets depend on the specific application and context of use .
Comparison with Similar Compounds
Similar Compounds
3,5-Dimethylaniline: Lacks the pyridine ring, making it less versatile in certain applications.
N-(Pyridin-4-ylmethyl)aniline: Lacks the methyl groups, which can affect its reactivity and properties.
4-Methyl-N-(pyridin-4-ylmethyl)aniline: Has only one methyl group, leading to different steric and electronic effects.
Uniqueness
3,5-Dimethyl-N-(pyridin-4-ylmethyl)aniline is unique due to the presence of both the pyridine ring and the two methyl groups, which confer specific reactivity and properties that are advantageous in various research and industrial applications .
Properties
IUPAC Name |
3,5-dimethyl-N-(pyridin-4-ylmethyl)aniline |
Source
|
---|---|---|
Source | PubChem | |
URL | https://pubchem.ncbi.nlm.nih.gov | |
Description | Data deposited in or computed by PubChem | |
InChI |
InChI=1S/C14H16N2/c1-11-7-12(2)9-14(8-11)16-10-13-3-5-15-6-4-13/h3-9,16H,10H2,1-2H3 |
Source
|
Source | PubChem | |
URL | https://pubchem.ncbi.nlm.nih.gov | |
Description | Data deposited in or computed by PubChem | |
InChI Key |
JQBCBNLHLZSPHS-UHFFFAOYSA-N |
Source
|
Source | PubChem | |
URL | https://pubchem.ncbi.nlm.nih.gov | |
Description | Data deposited in or computed by PubChem | |
Canonical SMILES |
CC1=CC(=CC(=C1)NCC2=CC=NC=C2)C |
Source
|
Source | PubChem | |
URL | https://pubchem.ncbi.nlm.nih.gov | |
Description | Data deposited in or computed by PubChem | |
Molecular Formula |
C14H16N2 |
Source
|
Source | PubChem | |
URL | https://pubchem.ncbi.nlm.nih.gov | |
Description | Data deposited in or computed by PubChem | |
DSSTOX Substance ID |
DTXSID001322021 |
Source
|
Record name | 3,5-dimethyl-N-(pyridin-4-ylmethyl)aniline | |
Source | EPA DSSTox | |
URL | https://comptox.epa.gov/dashboard/DTXSID001322021 | |
Description | DSSTox provides a high quality public chemistry resource for supporting improved predictive toxicology. | |
Molecular Weight |
212.29 g/mol |
Source
|
Source | PubChem | |
URL | https://pubchem.ncbi.nlm.nih.gov | |
Description | Data deposited in or computed by PubChem | |
Solubility |
24.8 [ug/mL] (The mean of the results at pH 7.4) |
Source
|
Record name | SID49643419 | |
Source | Burnham Center for Chemical Genomics | |
URL | https://pubchem.ncbi.nlm.nih.gov/bioassay/1996#section=Data-Table | |
Description | Aqueous solubility in buffer at pH 7.4 | |
CAS No. |
331970-83-3 |
Source
|
Record name | 3,5-dimethyl-N-(pyridin-4-ylmethyl)aniline | |
Source | EPA DSSTox | |
URL | https://comptox.epa.gov/dashboard/DTXSID001322021 | |
Description | DSSTox provides a high quality public chemistry resource for supporting improved predictive toxicology. | |
Disclaimer and Information on In-Vitro Research Products
Please be aware that all articles and product information presented on BenchChem are intended solely for informational purposes. The products available for purchase on BenchChem are specifically designed for in-vitro studies, which are conducted outside of living organisms. In-vitro studies, derived from the Latin term "in glass," involve experiments performed in controlled laboratory settings using cells or tissues. It is important to note that these products are not categorized as medicines or drugs, and they have not received approval from the FDA for the prevention, treatment, or cure of any medical condition, ailment, or disease. We must emphasize that any form of bodily introduction of these products into humans or animals is strictly prohibited by law. It is essential to adhere to these guidelines to ensure compliance with legal and ethical standards in research and experimentation.