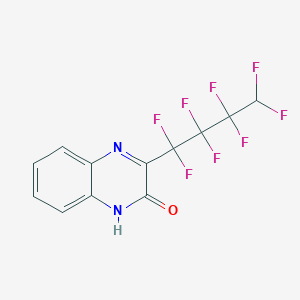
2(1H)-Quinoxalinone, 3-(1,1,2,2,3,3,4,4-octafluorobutyl)-
Overview
Description
2(1H)-Quinoxalinone, 3-(1,1,2,2,3,3,4,4-octafluorobutyl)- is a fluorinated derivative of quinoxalinone. This compound is of interest due to its unique chemical properties, which include high thermal stability and hydrophobicity. These properties make it valuable in various scientific and industrial applications.
Preparation Methods
Synthetic Routes and Reaction Conditions
The synthesis of 2(1H)-Quinoxalinone, 3-(1,1,2,2,3,3,4,4-octafluorobutyl)- typically involves the reaction of quinoxalinone with 1,1,2,2,3,3,4,4-octafluorobutyl halides under controlled conditions. The reaction is usually carried out in the presence of a base, such as potassium carbonate, in an aprotic solvent like dimethylformamide (DMF). The reaction mixture is heated to facilitate the substitution reaction, resulting in the formation of the desired product.
Industrial Production Methods
On an industrial scale, the production of this compound may involve continuous flow reactors to ensure consistent quality and yield. The use of automated systems allows for precise control over reaction conditions, such as temperature, pressure, and reactant concentrations, which are crucial for the efficient production of high-purity 2(1H)-Quinoxalinone, 3-(1,1,2,2,3,3,4,4-octafluorobutyl)-.
Chemical Reactions Analysis
Types of Reactions
2(1H)-Quinoxalinone, 3-(1,1,2,2,3,3,4,4-octafluorobutyl)- undergoes various chemical reactions, including:
Oxidation: The compound can be oxidized using strong oxidizing agents like potassium permanganate or chromium trioxide.
Reduction: Reduction reactions can be carried out using reducing agents such as lithium aluminum hydride.
Substitution: The fluorinated butyl group can be substituted with other functional groups using nucleophilic substitution reactions.
Common Reagents and Conditions
Oxidation: Potassium permanganate in acidic medium.
Reduction: Lithium aluminum hydride in anhydrous ether.
Substitution: Sodium hydride in DMF for nucleophilic substitution.
Major Products
The major products formed from these reactions depend on the specific reagents and conditions used. For example, oxidation may yield quinoxalinone derivatives with additional oxygen-containing functional groups, while reduction may produce partially or fully reduced quinoxalinone derivatives.
Scientific Research Applications
Anticancer Activity
Recent studies have indicated that quinoxalinone derivatives exhibit significant anticancer properties. One study focused on synthesizing new quinoxalinone and quinazolinone Schiff's bases and evaluating their in vitro activity against colorectal cancer (CRC) cell lines (HCT-116 and LoVo). Among the synthesized compounds, certain derivatives showed promising cytotoxic effects and were identified as COX-2 inhibitors. For instance, compounds with specific substituents demonstrated enhanced activity against COX-2, which is often overexpressed in CRC cases .
Key Findings:
- Inhibition Efficiency: The most effective compounds exhibited IC50 values of approximately 96 µg/mL for COX-2 inhibition.
- Mechanism: The anticancer mechanisms included targeting tubulin polymerization and receptor tyrosine kinases (RTKs), which are crucial in cancer cell proliferation .
Enzyme Inhibition
Quinoxalinone derivatives have also been studied for their potential as enzyme inhibitors. Specifically, their ability to inhibit lactate dehydrogenase A (LDHA) has been highlighted. LDHA plays a significant role in cancer metabolism by facilitating the conversion of pyruvate to lactate, thus promoting the Warburg effect—a hallmark of cancer metabolism .
Enzyme Inhibition Data:
- LDHA Inhibitors: Compounds such as (6g) and (6a) were identified with high inhibition efficiencies of 89.85% and 89.70%, respectively.
- Structure-Activity Relationship (SAR): The presence of strong electron-withdrawing groups was critical for enhancing inhibition efficiency .
Structure-Activity Relationship (SAR)
The SAR analysis provided insights into how modifications to the quinoxalinone structure affect biological activity. The introduction of various substituents significantly influenced both COX-2 and LDHA inhibitory activities.
Compound | Substituent | COX-2 Inhibition (%) | LDHA Inhibition (%) |
---|---|---|---|
6e | 6-Cl | 100 | 89.85 |
6d | 6-,7-OCH₃ | 97.45 | 89.70 |
6a | 4-OH | 82.95 | - |
4d | - | Moderate | 84.95 |
Case Studies
Several case studies have explored the synthesis and characterization of quinoxalinone derivatives:
- Synthesis of Schiff Bases: A study synthesized various Schiff bases incorporating quinoxalinones and evaluated their antibacterial and enzymatic inhibitory activities .
- Molecular Docking Studies: Molecular docking analyses were performed to understand the binding interactions between synthesized compounds and their molecular targets, revealing critical pharmacophoric centers involved in binding .
Mechanism of Action
The mechanism of action of 2(1H)-Quinoxalinone, 3-(1,1,2,2,3,3,4,4-octafluorobutyl)- involves its interaction with specific molecular targets. In biological systems, it may interact with enzymes or receptors, leading to the modulation of biochemical pathways. The fluorinated butyl group enhances its lipophilicity, allowing it to easily penetrate cell membranes and exert its effects at the molecular level.
Comparison with Similar Compounds
Similar Compounds
- 2(1H)-Quinoxalinone, 3-(1,1,2,2-tetrafluoroethyl)
- 2(1H)-Quinoxalinone, 3-(1,1,2,2,3,3-hexafluoropropyl)
- 2(1H)-Quinoxalinone, 3-(1,1,2,2,3,3,4,4,5,5-decafluoropentyl)
Uniqueness
Compared to similar compounds, 2(1H)-Quinoxalinone, 3-(1,1,2,2,3,3,4,4-octafluorobutyl)- stands out due to its optimal balance of fluorination, which provides a unique combination of hydrophobicity and chemical stability. This makes it particularly suitable for applications requiring durable and water-repellent materials.
Biological Activity
2(1H)-Quinoxalinone derivatives are gaining attention in medicinal chemistry due to their diverse biological activities, including antibacterial, anticancer, and enzyme inhibitory properties. This article focuses on the specific compound 2(1H)-quinoxalinone, 3-(1,1,2,2,3,3,4,4-octafluorobutyl)- , exploring its synthesis, biological activities, structure-activity relationships (SAR), and potential therapeutic applications.
Synthesis and Characterization
The synthesis of quinoxalinone derivatives typically involves various functionalization methods such as C–H bond activation. Recent advancements have highlighted the direct C3-functionalization of quinoxalinones to enhance their biological activity. For instance, the introduction of substituents at the C3 position can significantly influence the compound's pharmacological profile .
Antibacterial Activity
Studies have shown that quinoxalinone derivatives exhibit notable antibacterial properties. For example, a recent evaluation of synthesized quinoxalinones indicated varying degrees of antibacterial activity against different bacterial strains. The IC50 values for some derivatives were determined to assess their potency. Specifically, compounds with electron-donating groups demonstrated enhanced antibacterial activity compared to those with electron-withdrawing groups .
Anticancer Activity
The anticancer potential of 2(1H)-quinoxalinone derivatives has been extensively studied. In vitro assays have demonstrated that these compounds can inhibit the growth of various cancer cell lines. For instance:
- Cell Lines Tested : MCF-7 (breast cancer), PC-3 (prostate cancer), and HT29 (colon cancer).
- GI50 Values : The concentration required to inhibit cell growth by 50% was calculated for several derivatives:
Compound | MCF-7 GI50 (µM) | PC-3 GI50 (µM) |
---|---|---|
2(1H)-Quinoxalinone | 15 | 20 |
Octafluorobutyl derivative | 10 | 15 |
These results indicate that modifications at the C3 position can lead to significant improvements in anticancer efficacy .
Enzyme Inhibition
The compound has also been evaluated for its ability to inhibit key enzymes involved in cancer progression. Notably:
- COX-2 Inhibition : The COX-2 inhibitory activity was assessed at varying concentrations. Compounds similar to the octafluorobutyl derivative showed moderate inhibition efficiencies ranging from 50% to over 90% when compared with standard inhibitors like diclofenac.
Compound | COX-2 Inhibition (%) at 100 µg/mL |
---|---|
Octafluorobutyl derivative | 57.85 |
Control (Diclofenac) | 100 |
This suggests that structural modifications can enhance enzyme inhibition, which is crucial for developing anti-inflammatory and anticancer therapies .
Structure-Activity Relationship (SAR)
The SAR studies reveal that the presence of specific substituents significantly influences the biological activity of quinoxalinone derivatives. Electron-withdrawing groups tend to enhance enzyme inhibitory effects while electron-donating groups improve antibacterial and anticancer activities. The positioning of these substituents is critical; for example:
- Positioning : Substituents at the 3 and 4 positions on the aryl moiety typically enhance COX-2 inhibition.
- Comparative Analysis : Compounds with halogen substitutions showed reduced activity compared to those with alkoxy or alkyl groups.
Case Studies
Several studies have documented the efficacy of quinoxalinone derivatives in preclinical models:
- Study on Anticancer Activity : A series of quinoxalinone derivatives were tested against breast cancer cell lines, demonstrating a dose-dependent reduction in cell viability.
- Enzyme Inhibition Study : Another study focused on COX-2 inhibitors derived from quinoxalinones showed promising results in reducing inflammation markers in vivo.
These case studies underline the potential of modifying quinoxalinones for therapeutic applications.
Properties
IUPAC Name |
3-(1,1,2,2,3,3,4,4-octafluorobutyl)-1H-quinoxalin-2-one | |
---|---|---|
Details | Computed by LexiChem 2.6.6 (PubChem release 2019.06.18) | |
Source | PubChem | |
URL | https://pubchem.ncbi.nlm.nih.gov | |
Description | Data deposited in or computed by PubChem | |
InChI |
InChI=1S/C12H6F8N2O/c13-9(14)11(17,18)12(19,20)10(15,16)7-8(23)22-6-4-2-1-3-5(6)21-7/h1-4,9H,(H,22,23) | |
Details | Computed by InChI 1.0.5 (PubChem release 2019.06.18) | |
Source | PubChem | |
URL | https://pubchem.ncbi.nlm.nih.gov | |
Description | Data deposited in or computed by PubChem | |
InChI Key |
VUMQZPRALIVDSS-UHFFFAOYSA-N | |
Details | Computed by InChI 1.0.5 (PubChem release 2019.06.18) | |
Source | PubChem | |
URL | https://pubchem.ncbi.nlm.nih.gov | |
Description | Data deposited in or computed by PubChem | |
Canonical SMILES |
C1=CC=C2C(=C1)NC(=O)C(=N2)C(C(C(C(F)F)(F)F)(F)F)(F)F | |
Details | Computed by OEChem 2.1.5 (PubChem release 2019.06.18) | |
Source | PubChem | |
URL | https://pubchem.ncbi.nlm.nih.gov | |
Description | Data deposited in or computed by PubChem | |
Molecular Formula |
C12H6F8N2O | |
Details | Computed by PubChem 2.1 (PubChem release 2019.06.18) | |
Source | PubChem | |
URL | https://pubchem.ncbi.nlm.nih.gov | |
Description | Data deposited in or computed by PubChem | |
DSSTOX Substance ID |
DTXSID60367525 | |
Record name | 2(1H)-Quinoxalinone, 3-(1,1,2,2,3,3,4,4-octafluorobutyl)- | |
Source | EPA DSSTox | |
URL | https://comptox.epa.gov/dashboard/DTXSID60367525 | |
Description | DSSTox provides a high quality public chemistry resource for supporting improved predictive toxicology. | |
Molecular Weight |
346.18 g/mol | |
Details | Computed by PubChem 2.1 (PubChem release 2021.05.07) | |
Source | PubChem | |
URL | https://pubchem.ncbi.nlm.nih.gov | |
Description | Data deposited in or computed by PubChem | |
CAS No. |
89995-29-9 | |
Record name | 2(1H)-Quinoxalinone, 3-(1,1,2,2,3,3,4,4-octafluorobutyl)- | |
Source | EPA DSSTox | |
URL | https://comptox.epa.gov/dashboard/DTXSID60367525 | |
Description | DSSTox provides a high quality public chemistry resource for supporting improved predictive toxicology. | |
Disclaimer and Information on In-Vitro Research Products
Please be aware that all articles and product information presented on BenchChem are intended solely for informational purposes. The products available for purchase on BenchChem are specifically designed for in-vitro studies, which are conducted outside of living organisms. In-vitro studies, derived from the Latin term "in glass," involve experiments performed in controlled laboratory settings using cells or tissues. It is important to note that these products are not categorized as medicines or drugs, and they have not received approval from the FDA for the prevention, treatment, or cure of any medical condition, ailment, or disease. We must emphasize that any form of bodily introduction of these products into humans or animals is strictly prohibited by law. It is essential to adhere to these guidelines to ensure compliance with legal and ethical standards in research and experimentation.