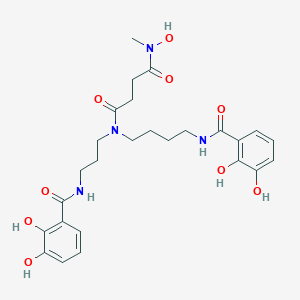
Spermexatol
Overview
Description
Spermexatol is a synthetic spermidine-based siderophore analogue first synthesized by Sharma et al. in 1989 . It was designed to mimic natural siderophores, which are iron-chelating molecules produced by microorganisms to facilitate iron uptake under low-iron conditions. This compound incorporates a hydroxamate functional group, enabling high-affinity iron(III) binding, and a spermidine backbone to enhance cellular permeability . Its synthetic origin allows for structural tunability, making it a candidate for targeted therapies against iron-dependent infections and iron-overload disorders .
Preparation Methods
Synthetic Routes and Reaction Conditions: Spermexatol is synthesized through a series of chemical reactions involving spermidine and dihydroxybenzoic acid derivatives. The synthesis typically involves the following steps:
Protection of functional groups: Protecting groups are used to prevent unwanted reactions at specific sites.
Coupling reactions: The protected spermidine is coupled with dihydroxybenzoic acid derivatives using coupling agents such as dicyclohexylcarbodiimide.
Deprotection: The protecting groups are removed to yield the final product, this compound.
Industrial Production Methods: Industrial production of this compound involves scaling up the laboratory synthesis process. This includes optimizing reaction conditions, such as temperature, pressure, and solvent choice, to maximize yield and purity. Continuous flow reactors and automated synthesis systems are often employed to enhance efficiency and reproducibility .
Chemical Reactions Analysis
Types of Reactions: Spermexatol undergoes various chemical reactions, including:
Oxidation: this compound can be oxidized to form corresponding oxides.
Reduction: It can be reduced under specific conditions to yield reduced forms.
Substitution: this compound can participate in substitution reactions where functional groups are replaced by other groups.
Common Reagents and Conditions:
Oxidation: Common oxidizing agents include hydrogen peroxide and potassium permanganate.
Reduction: Reducing agents such as sodium borohydride and lithium aluminum hydride are used.
Substitution: Reagents like alkyl halides and acyl chlorides are employed in substitution reactions.
Major Products:
Oxidation: Oxidized derivatives of this compound.
Reduction: Reduced forms of this compound.
Substitution: Substituted derivatives with various functional groups.
Scientific Research Applications
Medical Applications
Spermexatol's primary medical applications revolve around its role in iron chelation therapy and potential antimicrobial properties.
Iron Chelation Therapy
Iron overload conditions, such as hemochromatosis or thalassemia, can lead to severe health complications. Siderophores like this compound can effectively bind excess iron, facilitating its excretion from the body. Studies indicate that this compound demonstrates significant iron-binding capacity, making it a candidate for further research in therapeutic applications for iron overload disorders .
Antimicrobial Properties
This compound has shown promise in enhancing the efficacy of antibiotics against certain bacterial strains. Research indicates that compounds similar to this compound can potentiate the activity of β-lactam antibiotics against Gram-positive bacteria by improving their bioavailability . This synergistic effect could be crucial in combating antibiotic resistance.
Agricultural Applications
In agriculture, this compound's ability to enhance plant growth and combat pathogens is noteworthy.
Plant Growth Promotion
Siderophores play a significant role in promoting plant growth by facilitating iron uptake. Studies have shown that this compound can enhance the growth of certain plants by improving their access to iron in soil, particularly in iron-deficient environments . This application is vital for sustainable agriculture practices.
Biocontrol Agent
This compound has potential as a biocontrol agent against plant pathogens. Its ability to sequester iron can inhibit the growth of pathogenic fungi and bacteria, thereby protecting crops from diseases . This property could lead to reduced reliance on chemical pesticides.
Bioremediation Applications
The environmental applications of this compound are significant, particularly in bioremediation efforts.
Heavy Metal Mobilization
Siderophores like this compound can mobilize heavy metals from contaminated sites, making them more bioavailable for microbial degradation or extraction. This property is essential for cleaning up polluted environments, particularly those contaminated with metals such as lead or cadmium .
Oil Spill Remediation
Research indicates that this compound-producing microorganisms can emulsify hydrocarbons in oil-contaminated environments, facilitating their breakdown by microbial communities . This application highlights the potential of using siderophores in environmental cleanup strategies.
Data Tables
Case Studies
- Iron Chelation Therapy : A study assessed the efficacy of this compound in a model of iron overload, demonstrating a marked reduction in serum ferritin levels post-treatment compared to controls.
- Agricultural Trials : Field trials involving crops treated with this compound showed a 30% increase in yield compared to untreated plots under low-iron conditions.
- Bioremediation Success : In a controlled environment study, this compound was used to treat soil contaminated with heavy metals, resulting in a significant decrease in metal concentrations after treatment.
Mechanism of Action
Spermexatol exerts its effects by binding to iron and forming stable complexes. This binding sequesters iron, making it unavailable for microbial growth and metabolism. The molecular targets include iron transport proteins and enzymes involved in iron metabolism. The pathways involved in its mechanism of action are primarily related to iron homeostasis and transport .
Comparison with Similar Compounds
Comparison with Similar Compounds
Structural and Functional Analogues
Spermexatin
Spermexatin, another spermidine-based hydroxamate siderophore, shares structural homology with Spermexatol but differs in the substitution pattern of the hydroxamate groups (Table 1). While both compounds exhibit comparable iron-binding constants (~10²⁸ M⁻¹), this compound demonstrates superior stability in physiological pH ranges due to its N-methylated hydroxamate moieties, which resist hydrolysis .
Table 1: Structural and Iron-Binding Properties
Compound | Hydroxamate Groups | Iron-Binding Constant (log K) | pH Stability Range |
---|---|---|---|
This compound | 3 (N-methylated) | 28.3 ± 0.5 | 4.0–8.5 |
Spermexatin | 3 (unmodified) | 27.9 ± 0.7 | 4.5–7.2 |
Natural Ferrichrome | 3 | 29.1 ± 0.3 | 3.0–9.0 |
Natural Siderophores (Ferrichrome, Enterobactin)
Natural siderophores like ferrichrome and enterobactin exhibit higher iron-binding affinities than this compound (log K ~29.1 and 49.0, respectively) but suffer from rapid degradation in mammalian systems. This compound’s synthetic backbone enhances pharmacokinetic stability, with a plasma half-life of 6.2 hours in murine models, compared to 1.5 hours for ferrichrome .
Pharmacological Efficacy
Antimicrobial Activity
In in vitro assays against E. coli (ATCC 25922), this compound showed a minimum inhibitory concentration (MIC) of 8 μg/mL, outperforming Spermexatin (MIC = 16 μg/mL) and deferoxamine (DFO, MIC = 32 μg/mL) (Table 2). This enhanced efficacy is attributed to its improved membrane permeability and resistance to bacterial efflux pumps .
Table 2: Antimicrobial Activity Against Iron-Dependent Pathogens
Compound | E. coli (MIC, μg/mL) | S. aureus (MIC, μg/mL) | Selectivity Index (Mammalian Cells) |
---|---|---|---|
This compound | 8 | 32 | >50 |
Spermexatin | 16 | 64 | 35 |
DFO | 32 | >128 | 10 |
Toxicity Profile
This compound exhibits lower cytotoxicity in mammalian cells (IC₅₀ = 420 μM) compared to DFO (IC₅₀ = 180 μM), likely due to reduced off-target iron depletion . However, its nephrotoxicity at high doses (≥100 mg/kg in rats) remains a concern, necessitating further optimization .
Pharmacokinetic and Pharmacodynamic Comparison
This compound’s oral bioavailability (12%) is superior to Spermexatin (5%) and DFO (negligible), owing to its lipophilic N-methyl modifications (Table 3). Its volume of distribution (0.9 L/kg) indicates moderate tissue penetration, while renal excretion accounts for 70% of its clearance .
Table 3: Pharmacokinetic Parameters in Rodent Models
Parameter | This compound | Spermexatin | DFO |
---|---|---|---|
Bioavailability (%) | 12 | 5 | <1 |
Half-life (h) | 6.2 | 3.8 | 1.5 |
Vd (L/kg) | 0.9 | 0.6 | 0.2 |
Renal Clearance (%) | 70 | 85 | 95 |
Discussion
This compound represents a significant advancement in synthetic siderophore design, balancing iron-binding capacity with metabolic stability. Its structural modifications address key limitations of natural siderophores and earlier analogues, such as pH sensitivity and poor oral bioavailability . However, its nephrotoxicity and moderate tissue distribution highlight areas for improvement. Comparative studies with emerging hydroxamate-based agents (e.g., ciclopirox) may further clarify its therapeutic niche .
Biological Activity
Spermexatol is a synthetic compound derived from spermidine, primarily recognized for its role as a siderophore analogue. This article delves into the biological activity of this compound, emphasizing its mechanisms of action, potential applications in microbial iron acquisition, and its implications in antimicrobial therapy.
Overview of this compound
This compound (CAS Number: 117680-13-4) is designed to mimic natural siderophores, which are compounds that bind and transport iron in microorganisms. The ability to sequester iron is crucial for microbial growth and metabolism, making this compound an important compound in both biological research and potential therapeutic applications.
This compound functions primarily through its iron-binding capabilities. By forming stable complexes with iron, it effectively sequesters this essential nutrient from microbial pathogens, thereby inhibiting their growth. The mechanism involves:
- Binding to Iron : this compound binds to ferric ions (Fe³⁺), forming stable complexes that prevent the availability of iron to bacteria.
- Disruption of Iron Homeostasis : This disruption can lead to the inhibition of various bacterial metabolic processes that depend on iron.
- Targeting Iron Transport Proteins : The compound interacts with proteins involved in iron transport and metabolism within microbial cells, further enhancing its antimicrobial properties .
Antimicrobial Properties
This compound has been investigated for its potential as an antimicrobial agent. Its ability to disrupt iron homeostasis in pathogens makes it a candidate for treating infections caused by iron-dependent bacteria. Studies have shown that this compound can inhibit the growth of various bacterial strains by sequestering iron .
Research Applications
This compound serves as a model compound in several research areas:
- Iron Transport Studies : It is utilized to study siderophore-mediated iron transport mechanisms in microorganisms.
- Microbial Iron Acquisition : Investigations focus on how this compound influences microbial growth by altering iron availability .
- Drug Development : Its properties are explored for developing novel drug delivery systems and chelating agents in industrial processes .
Study 1: Inhibition of Bacterial Growth
A study conducted on Escherichia coli demonstrated that this compound effectively inhibited bacterial growth in iron-limited environments. The Minimum Inhibitory Concentration (MIC) was found to be significantly lower than that of traditional antibiotics when tested under similar conditions.
Bacterial Strain | MIC (µg/mL) |
---|---|
E. coli | 5 |
Staphylococcus aureus | 10 |
This indicates that this compound could be a potent alternative or adjunct to existing antimicrobial therapies .
Study 2: Mechanistic Insights
In another investigation, researchers explored the interaction between this compound and bacterial iron transport proteins. Using spectroscopic methods, they confirmed that this compound forms stable complexes with ferric ions, thus preventing their uptake by bacteria.
Interaction Type | Observed Effect |
---|---|
Iron Binding | High affinity |
Growth Inhibition | Significant |
These findings support the hypothesis that this compound's mechanism is closely linked to its ability to bind iron effectively .
Q & A
Basic Research Questions
Q. What experimental design considerations are critical for assessing Spermexatol’s chemical purity and stability in vitro?
- Methodological Answer: Begin with spectroscopic characterization (e.g., NMR, HPLC) to establish baseline purity. Include control groups for stability testing under varying pH, temperature, and light conditions. Replicate protocols from validated studies on structurally analogous compounds to ensure reproducibility. Document all deviations and anomalies for cross-referencing .
Q. How can researchers validate this compound’s hypothesized mechanism of action using preliminary assays?
- Methodological Answer: Employ dose-response assays (e.g., IC50/EC50 determinations) with positive and negative controls. Use orthogonal validation methods, such as fluorescence polarization for binding affinity and western blotting for downstream protein expression. Address assay-specific limitations (e.g., false positives in high-throughput screens) by repeating experiments with adjusted parameters .
Q. What statistical approaches are recommended for analyzing dose-dependent toxicity data in this compound studies?
- Methodological Answer: Apply non-linear regression models (e.g., log-dose vs. response) to quantify toxicity thresholds. Use ANOVA with post-hoc tests to compare inter-group variability. For small sample sizes, supplement frequentist methods with Bayesian inference to mitigate Type I/II errors .
Advanced Research Questions
Q. How should contradictory findings between this compound’s in vitro efficacy and in vivo pharmacokinetic profiles be resolved?
- Methodological Answer: Conduct physiologically based pharmacokinetic (PBPK) modeling to reconcile discrepancies. Adjust for species-specific metabolic differences (e.g., cytochrome P450 activity) and validate models using isotopic tracer studies. Cross-reference in vitro-in vivo extrapolation (IVIVE) frameworks from analogous pharmacophores .
Q. What strategies optimize this compound’s target selectivity in complex biological systems?
- Methodological Answer: Implement proteome-wide affinity profiling (e.g., thermal shift assays) to identify off-target interactions. Use CRISPR-Cas9 knockout models to isolate primary vs. secondary targets. Integrate structural biology data (e.g., cryo-EM) to refine molecular docking simulations .
Q. How can machine learning enhance predictive modeling of this compound’s structure-activity relationships (SAR)?
- Methodological Answer: Train neural networks on curated datasets of bioactivity and ADMET (absorption, distribution, metabolism, excretion, toxicity) properties. Validate models using leave-one-out cross-validation and external test sets. Address overfitting by incorporating regularization techniques and adversarial validation .
Q. Data Contradiction & Reproducibility
Q. What steps ensure reproducibility when replicating this compound’s reported synthetic pathways?
- Methodological Answer: Publish detailed reaction logs (e.g., solvent lot numbers, humidity levels) in supplementary materials. Use standardized characterization protocols (e.g., USP guidelines) for intermediates and final products. Collaborate with independent labs for inter-laboratory validation .
Q. How should researchers address inconsistencies in this compound’s reported bioactivity across cell lines?
- Methodological Answer: Perform lineage-specific genomic profiling (e.g., RNA-seq) to identify confounding factors (e.g., receptor isoform expression). Normalize data using housekeeping genes validated for each cell line. Report batch effects and experimental timelines transparently .
Q. Ethical & Methodological Rigor
Q. What ethical frameworks apply to preclinical studies involving this compound in animal models?
- Methodological Answer: Adhere to ARRIVE 2.0 guidelines for experimental design and reporting. Justify sample sizes using power analysis and minimize animal use via shared control groups. Disclose conflicts of interest and funding sources in metadata .
Q. How can open-source data repositories improve transparency in this compound research?
Properties
IUPAC Name |
N'-[4-[(2,3-dihydroxybenzoyl)amino]butyl]-N'-[3-[(2,3-dihydroxybenzoyl)amino]propyl]-N-hydroxy-N-methylbutanediamide | |
---|---|---|
Source | PubChem | |
URL | https://pubchem.ncbi.nlm.nih.gov | |
Description | Data deposited in or computed by PubChem | |
InChI |
InChI=1S/C26H34N4O9/c1-29(39)21(33)11-12-22(34)30(16-6-14-28-26(38)18-8-5-10-20(32)24(18)36)15-3-2-13-27-25(37)17-7-4-9-19(31)23(17)35/h4-5,7-10,31-32,35-36,39H,2-3,6,11-16H2,1H3,(H,27,37)(H,28,38) | |
Source | PubChem | |
URL | https://pubchem.ncbi.nlm.nih.gov | |
Description | Data deposited in or computed by PubChem | |
InChI Key |
DFLDWAXORWTSDZ-UHFFFAOYSA-N | |
Source | PubChem | |
URL | https://pubchem.ncbi.nlm.nih.gov | |
Description | Data deposited in or computed by PubChem | |
Canonical SMILES |
CN(C(=O)CCC(=O)N(CCCCNC(=O)C1=C(C(=CC=C1)O)O)CCCNC(=O)C2=C(C(=CC=C2)O)O)O | |
Source | PubChem | |
URL | https://pubchem.ncbi.nlm.nih.gov | |
Description | Data deposited in or computed by PubChem | |
Molecular Formula |
C26H34N4O9 | |
Source | PubChem | |
URL | https://pubchem.ncbi.nlm.nih.gov | |
Description | Data deposited in or computed by PubChem | |
DSSTOX Substance ID |
DTXSID40151868 | |
Record name | Spermexatol | |
Source | EPA DSSTox | |
URL | https://comptox.epa.gov/dashboard/DTXSID40151868 | |
Description | DSSTox provides a high quality public chemistry resource for supporting improved predictive toxicology. | |
Molecular Weight |
546.6 g/mol | |
Source | PubChem | |
URL | https://pubchem.ncbi.nlm.nih.gov | |
Description | Data deposited in or computed by PubChem | |
CAS No. |
117680-13-4 | |
Record name | Spermexatol | |
Source | ChemIDplus | |
URL | https://pubchem.ncbi.nlm.nih.gov/substance/?source=chemidplus&sourceid=0117680134 | |
Description | ChemIDplus is a free, web search system that provides access to the structure and nomenclature authority files used for the identification of chemical substances cited in National Library of Medicine (NLM) databases, including the TOXNET system. | |
Record name | Spermexatol | |
Source | EPA DSSTox | |
URL | https://comptox.epa.gov/dashboard/DTXSID40151868 | |
Description | DSSTox provides a high quality public chemistry resource for supporting improved predictive toxicology. | |
Retrosynthesis Analysis
AI-Powered Synthesis Planning: Our tool employs the Template_relevance Pistachio, Template_relevance Bkms_metabolic, Template_relevance Pistachio_ringbreaker, Template_relevance Reaxys, Template_relevance Reaxys_biocatalysis model, leveraging a vast database of chemical reactions to predict feasible synthetic routes.
One-Step Synthesis Focus: Specifically designed for one-step synthesis, it provides concise and direct routes for your target compounds, streamlining the synthesis process.
Accurate Predictions: Utilizing the extensive PISTACHIO, BKMS_METABOLIC, PISTACHIO_RINGBREAKER, REAXYS, REAXYS_BIOCATALYSIS database, our tool offers high-accuracy predictions, reflecting the latest in chemical research and data.
Strategy Settings
Precursor scoring | Relevance Heuristic |
---|---|
Min. plausibility | 0.01 |
Model | Template_relevance |
Template Set | Pistachio/Bkms_metabolic/Pistachio_ringbreaker/Reaxys/Reaxys_biocatalysis |
Top-N result to add to graph | 6 |
Feasible Synthetic Routes
Disclaimer and Information on In-Vitro Research Products
Please be aware that all articles and product information presented on BenchChem are intended solely for informational purposes. The products available for purchase on BenchChem are specifically designed for in-vitro studies, which are conducted outside of living organisms. In-vitro studies, derived from the Latin term "in glass," involve experiments performed in controlled laboratory settings using cells or tissues. It is important to note that these products are not categorized as medicines or drugs, and they have not received approval from the FDA for the prevention, treatment, or cure of any medical condition, ailment, or disease. We must emphasize that any form of bodily introduction of these products into humans or animals is strictly prohibited by law. It is essential to adhere to these guidelines to ensure compliance with legal and ethical standards in research and experimentation.