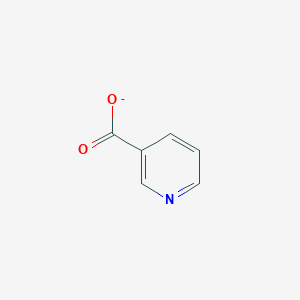
Nicotinate
Overview
Description
Nicotinate, also known as nicotinic acid or vitamin B3, is a water-soluble compound critical in cellular metabolism as a precursor to nicotinamide adenine dinucleotide (NAD+) and nicotinamide adenine dinucleotide phosphate (NADP+), coenzymes essential for redox reactions and energy production . It is widely used pharmacologically to treat dyslipidemia due to its ability to lower LDL cholesterol and triglycerides while raising HDL cholesterol . Structurally, this compound consists of a pyridine ring with a carboxylic acid group at the 3-position (C₅H₄N–COOH). Its derivatives, such as esters (e.g., hexyl this compound, menthyl this compound) and metal complexes (e.g., chromium(III) this compound), exhibit distinct physicochemical and biological properties .
Q & A
Q. Basic: What are the standard analytical methods for quantifying nicotinate levels in biological matrices, and how can reproducibility be ensured?
Answer:
this compound quantification typically employs liquid chromatography-mass spectrometry (LC-MS) or high-performance liquid chromatography (HPLC) with UV detection. Key methodological considerations include:
- Sample preparation : Acidic extraction to stabilize this compound and remove interfering compounds.
- Validation : Calibration curves, spike-recovery tests, and intra-/inter-day precision assessments to meet analytical standards .
- Reproducibility : Detailed protocols for instrument settings (e.g., column type, mobile phase gradients) and adherence to guidelines like NIH reporting standards for preclinical studies to enable replication .
Q. Advanced: How can structural determinants of this compound phosphoribosyltransferase (NAPRT) activity be investigated across species with divergent enzyme architectures?
Answer:
Comparative studies require:
- X-ray crystallography : Resolve 3D structures of NAPRT from thermophilic (e.g., Thermoplasma acidophilum) and mesophilic species to identify conserved active-site residues .
- Site-directed mutagenesis : Target β-strands and helices in the C-terminal domain to assess catalytic contributions.
- Kinetic assays : Measure PRPP binding affinity (Km) and turnover rates (kcat) under varying pH/temperature conditions to correlate structure-function relationships .
Q. Basic: What experimental designs are optimal for studying this compound’s role in cellular NAD+ biosynthesis?
Answer:
- Isotopic labeling : Use <sup>13</sup>C-nicotinate to track incorporation into NAD+ via LC-MS.
- Knockdown models : siRNA targeting NAPRT to observe NAD+ depletion effects.
- Controls : Include nicotinamide-treated groups to distinguish salvage pathway contributions .
Q. Advanced: How can metabolomics data on this compound metabolism be integrated with transcriptomic profiles to identify regulatory nodes?
Answer:
- Multi-omics pipelines : Pair LC-MS-based this compound quantification with RNA-seq to correlate metabolite levels with NAPRT gene expression.
- Pathway enrichment : Tools like MetaboAnalyst 5.0 map this compound-related DEMs (differentially expressed metabolites) to lipid metabolism pathways (e.g., sphingolipid metabolism) and cross-reference with KEGG transcriptomic datasets .
- Network analysis : Use weighted gene co-expression networks (WGCNA) to identify hub genes regulating NAD+ synthesis .
Q. Basic: What are common pitfalls in assessing this compound’s bioavailability, and how can they be mitigated?
Answer:
- Matrix effects : Plasma proteins may bind this compound; use protein precipitation or solid-phase extraction.
- Interindividual variability : Stratify cohorts by age, diet, or gut microbiota composition.
- Dose-response calibration : Validate in vivo models (e.g., rodents) with controlled this compound administration routes (oral vs. intravenous) .
Q. Advanced: How can contradictions in this compound enzyme kinetics studies (e.g., conflicting Km values across species) be resolved?
Answer:
- Comparative docking studies : Model PRPP binding in NAPRT homologs using software like AutoDock Vina to identify species-specific binding pocket conformations .
- pH/Temperature profiling : Test enzyme activity under physiologically relevant conditions (e.g., thermophilic enzymes at 70°C).
- Meta-analysis : Aggregate kinetic data from public repositories (e.g., BRENDA) to identify outliers and normalize experimental parameters .
Q. Basic: How can researchers ensure reproducibility in this compound-related cell culture experiments?
Answer:
- Culture conditions : Standardize media (e.g., DMEM vs. RPMI), fetal bovine serum (FBS) batches, and cell passage numbers.
- Documentation : Publish full protocols in supplementary materials, including this compound stock preparation (solvent, concentration) and exposure duration .
- Data sharing : Deposit raw metabolomics data in repositories like MetaboLights for independent validation .
Q. Advanced: What strategies evaluate this compound’s epigenetic effects via NAD+-dependent mechanisms (e.g., sirtuin activation)?
Answer:
- ChIP-seq : Profile histone acetylation (H3K9ac) in sirtuin-overexpressing cells treated with this compound.
- Methylation analysis : Use bisulfite sequencing to assess DNA methylation changes linked to NAD+ availability.
- Pharmacological inhibition : Co-treat with sirtuin inhibitors (e.g., EX527) to isolate this compound-specific effects .
Comparison with Similar Compounds
Comparison with Structurally Similar Compounds
Nicotinate Esters
This compound esters (e.g., methyl this compound, hexyl this compound) are prodrugs designed to enhance lipid solubility and bioavailability. These esters are hydrolyzed by human serum albumin (HSA) or esterases in plasma to release free nicotinic acid. Key differences include:
- Hydrolysis Rates : Methyl this compound has a half-life of >95 hours in HSA, whereas 2-butoxyethyl this compound hydrolyzes rapidly (<15 minutes) .
- Pharmacological Effects : Hexyl this compound penetrates skin more effectively than nicotinic acid, making it useful in topical formulations for improving microcirculation .
- Thermal Stability : Menthyl this compound, synthesized via solvent-free methods, exhibits industrial viability for cosmetic applications due to its stability and purity .
Table 1: Hydrolysis Rates of this compound Esters in Human Serum Albumin (50 μM, pH 7.4, 37°C)
Ester | Half-Life (hours) | Reference |
---|---|---|
Methyl this compound | >95 | |
2-Butoxyethyl this compound | <0.25 | |
Hexyl this compound | Not reported |
Nicotinamide (Vitamin B3 Amide)
Nicotinamide (C₅H₄N–CONH₂) shares the pyridine core of this compound but replaces the carboxylic acid with an amide group. Key distinctions include:
Vitamin E this compound
α-Tocopheryl this compound combines nicotinic acid with α-tocopherol (vitamin E). Unlike α-tocopheryl succinate, it lacks anticancer activity and is hydrolyzed more slowly, leading to prolonged systemic exposure .
Table 2: Comparative Effects of Vitamin E Esters
Ester | Key Properties | Reference |
---|---|---|
α-Tocopheryl this compound | Slow hydrolysis; no anticancer activity | |
α-Tocopheryl succinate | Rapid hydrolysis; induces apoptosis |
Metal-Nicotinate Complexes
- Chromium(III) this compound : Used in nutritional supplements, but its polymeric structure (Cr³⁺-nicotinate-hydroxide-water) varies with synthesis methods, complicating clinical interpretations .
- Ni/Zn-Nicotinate Complexes : Employed in semiconductor photodiodes. Ni-centered this compound has a bandgap of 3.87 eV, while Zn-centered this compound exhibits 4.04 eV, influencing optoelectronic performance .
Pharmacodynamic and Kinetic Comparisons
- Xanthinol this compound: A xanthine derivative of this compound, it enhances cerebral oxygen supply and shows age-dependent efficacy in memory improvement (effective in elderly patients) compared to nicotinic acid, which benefits younger cohorts .
- Bacterial Metabolism: Azorhizobium caulinodans metabolizes this compound as a nitrogen source but cannot utilize analogs like 2-chlorothis compound or 2-aminothis compound, highlighting structural specificity .
Preparation Methods
Preparation of 2-Methyl Nicotinate via Acid-Catalyzed Reaction of Precursors
One of the most prominent methods involves a multi-step synthesis starting from readily available organic compounds, such as tetramethoxypropane derivatives, reacting under acid catalysis to produce intermediates that are subsequently transformed into 2-methyl this compound.
Methodology Overview:
- Initial reaction of tetramethoxypropane with hydrochloric acid at controlled temperatures (around 40°C) for approximately 3 hours, yielding a key intermediate (Compound B).
- Subsequent reaction of Compound B with β-aminocrotonate esters in an organic solvent at 50-60°C for 5-7 hours produces the 2-methyl this compound.
Research Findings:
- The process avoids the use of acrolein, a toxic and hazardous reagent, favoring safer reagents like tetramethoxy derivatives.
- Reaction conditions such as temperature (50-60°C) and reaction times (around 5-7 hours) are optimized to maximize yield and conversion efficiency.
- The overall yield of 2-methyl this compound can reach approximately 71%, with purity levels exceeding 98%, demonstrating the process's efficiency.
Data Table 1: Preparation Parameters and Outcomes
Step | Reagent/Reactant | Temperature (°C) | Reaction Time (hours) | Yield (%) | Purity (%) |
---|---|---|---|---|---|
1 | Tetramethoxypropane + Hydrochloric acid | 40 | 3 | - | - |
2 | Compound B + β-aminocrotonate ester | 50-60 | 5-7 | 71 | >98 |
Note: The process emphasizes the avoidance of acrolein, simplifying scale-up and reducing toxicity.
Alternative Synthesis Using Esterification and Transesterification
Another approach involves transesterification reactions where menthol reacts with nicotinic acid esters (such as methyl or ethyl this compound) in the presence of catalysts like sodium methoxide or potassium methoxide.
Methodology Overview:
- Menthol is reacted with methyl or ethyl this compound under alkaline catalysis at temperatures around 60°C.
- This process is often conducted under vacuum to facilitate distillation of excess reactants and by-products, leading to high-purity menthyl this compound.
Data Table 2: Transesterification Process Parameters
Reactants | Catalyst | Temperature (°C) | Reaction Time (hours) | Yield (%) | Purity (%) |
---|---|---|---|---|---|
Menthol + Methyl this compound | Sodium methoxide | 60 | 5-7 | 83-87 | >98.5 |
Note: The process benefits from continuous distillation under vacuum, which ensures high purity and minimizes secondary reactions.
Innovative Methods Using Activated Carbon in Distillation
Recent advancements have introduced the use of activated carbons during the distillation phase to improve the purity and yield of this compound compounds, especially for high-boiling compounds like menthyl this compound.
Methodology Overview:
- During vacuum distillation at temperatures around 150-170°C, powdered activated carbon is added in small quantities (0.3-1.2% by weight).
- The activated carbon acts as an inert adsorbent, removing residual impurities and preventing thermal degradation of the product.
Research Findings:
- This method yields menthyl this compound with purity levels greater than 99.5%, with yields around 83-87%.
- The process is environmentally friendly, as it eliminates the need for extensive washing or neutralization steps, and reduces solvent use.
Data Table 3: Activated Carbon-Assisted Distillation
Additive | Concentration (% w/w) | Temperature (°C) | Vacuum (mbar) | Yield (%) | Purity (%) |
---|---|---|---|---|---|
Powdered activated carbon | 0.3-1.2 | 150-170 | 0.5-2 | 83-87 | >99.5 |
Note: The inertness and thermal stability of activated carbons make them ideal for high-temperature distillation of this compound derivatives.
Environmental and Industrial Considerations
The described methods emphasize environmentally sustainable practices:
- Avoidance of hazardous reagents such as acrolein and methylene chloride.
- Minimal use of water and organic solvents, reducing waste and disposal issues.
- High yields and purities facilitate industrial scalability and cost efficiency.
Summary of Key Findings
Aspect | Details |
---|---|
Main Precursors | Tetramethoxypropane derivatives, nicotinic acid esters, menthol |
Catalysts | Acid catalysts (hydrochloric, p-toluenesulfonic acid), alkoxides (sodium/methyl) |
Reaction Conditions | Temperatures between 40-60°C, reaction times 3-7 hours |
Purification | Vacuum distillation, activated carbon adsorption |
Yields | Typically 71-87% |
Purity | Usually >98%, often exceeding 99.5% with activated carbon |
Properties
Molecular Formula |
C6H4NO2- |
---|---|
Molecular Weight |
122.1g/mol |
IUPAC Name |
pyridine-3-carboxylate |
InChI |
InChI=1S/C6H5NO2/c8-6(9)5-2-1-3-7-4-5/h1-4H,(H,8,9)/p-1 |
InChI Key |
PVNIIMVLHYAWGP-UHFFFAOYSA-M |
SMILES |
C1=CC(=CN=C1)C(=O)[O-] |
Canonical SMILES |
C1=CC(=CN=C1)C(=O)[O-] |
Synonyms |
3 Pyridinecarboxylic Acid 3-Pyridinecarboxylic Acid Aluminum Salt, Niacin Enduracin Hydrochloride, Niacin Induracin Lithium Nicotinate Niacin Niacin Aluminum Salt Niacin Ammonium Salt Niacin Calcium Salt Niacin Cobalt (2+) Salt Niacin Copper (2+) Salt Niacin Hydrochloride Niacin Iron (2+) Salt Niacin Lithium Salt Niacin Lithium Salt, Hemihydrate Niacin Magnesium Salt Niacin Manganese (2+) Salt Niacin Potassium Salt Niacin Sodium Salt Niacin Tartrate Niacin Tosylate Niacin Zinc Salt Nicamin Nico 400 Nico-400 Nico400 Nicobid Nicocap Nicolar Nicotinate Nicotinate, Lithium Nicotinic Acid Potassium Salt, Niacin Sodium Salt, Niacin Tartrate, Niacin Tosylate, Niacin Wampocap |
Origin of Product |
United States |
Synthesis routes and methods I
Procedure details
Synthesis routes and methods II
Procedure details
Retrosynthesis Analysis
AI-Powered Synthesis Planning: Our tool employs the Template_relevance Pistachio, Template_relevance Bkms_metabolic, Template_relevance Pistachio_ringbreaker, Template_relevance Reaxys, Template_relevance Reaxys_biocatalysis model, leveraging a vast database of chemical reactions to predict feasible synthetic routes.
One-Step Synthesis Focus: Specifically designed for one-step synthesis, it provides concise and direct routes for your target compounds, streamlining the synthesis process.
Accurate Predictions: Utilizing the extensive PISTACHIO, BKMS_METABOLIC, PISTACHIO_RINGBREAKER, REAXYS, REAXYS_BIOCATALYSIS database, our tool offers high-accuracy predictions, reflecting the latest in chemical research and data.
Strategy Settings
Precursor scoring | Relevance Heuristic |
---|---|
Min. plausibility | 0.01 |
Model | Template_relevance |
Template Set | Pistachio/Bkms_metabolic/Pistachio_ringbreaker/Reaxys/Reaxys_biocatalysis |
Top-N result to add to graph | 6 |
Feasible Synthetic Routes
Disclaimer and Information on In-Vitro Research Products
Please be aware that all articles and product information presented on BenchChem are intended solely for informational purposes. The products available for purchase on BenchChem are specifically designed for in-vitro studies, which are conducted outside of living organisms. In-vitro studies, derived from the Latin term "in glass," involve experiments performed in controlled laboratory settings using cells or tissues. It is important to note that these products are not categorized as medicines or drugs, and they have not received approval from the FDA for the prevention, treatment, or cure of any medical condition, ailment, or disease. We must emphasize that any form of bodily introduction of these products into humans or animals is strictly prohibited by law. It is essential to adhere to these guidelines to ensure compliance with legal and ethical standards in research and experimentation.