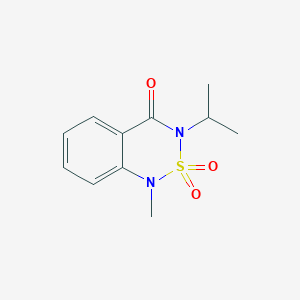
Bentazon methyl
Overview
Description
AMG925 is a dual inhibitor of Fms-like tyrosine kinase 3 and cyclin-dependent kinase 4. It has shown potential in overcoming resistance to Fms-like tyrosine kinase 3 inhibitors in the treatment of acute myeloid leukemia. This compound is currently in the late stages of preclinical development and has demonstrated significant antitumor activity in various models of acute myeloid leukemia .
Mechanism of Action
Target of Action
Bentazon methyl, also known as N-Methylbentazon, primarily targets the photosynthetic electron transport chain in plants . It specifically inhibits photosystem II (PSII), a key component of the photosynthetic process .
Mode of Action
The compound interacts with its target by causing an irreversible blockage of the photosynthetic electron transport . This blockage prevents the normal flow of electrons down the electron transport chain, disrupting the photosynthetic process. As a result, the plant’s ability to convert light energy into chemical energy is impaired, leading to a cessation of growth and eventual plant death .
Biochemical Pathways
The primary biochemical pathway affected by this compound is photosynthesis. By inhibiting PSII, the compound disrupts the light-dependent reactions of photosynthesis, which are crucial for the production of ATP and NADPH . These molecules are essential for the light-independent reactions (Calvin cycle), where carbon dioxide is fixed into glucose. Therefore, the inhibition of PSII indirectly affects the entire photosynthetic pathway .
Pharmacokinetics
It’s known that the compound is highly soluble in water , suggesting it could be readily absorbed and distributed within the plant system. The compound is metabolized in plants into two major metabolites, the glycosyl conjugates of 6- and 8-hydroxybentazon
Result of Action
The primary result of this compound’s action is the inhibition of photosynthesis, leading to growth cessation and eventual plant death . This effect is due to the disruption of the photosynthetic electron transport chain, which impairs the plant’s ability to convert light energy into chemical energy .
Action Environment
Environmental factors can influence the action, efficacy, and stability of this compound. For instance, the compound’s high water solubility suggests that it could be easily leached from the soil, potentially reducing its efficacy . Additionally, the compound may be persistent in water under certain conditions
Biochemical Analysis
Biochemical Properties
Bentazon Methyl interacts with various enzymes and proteins. It inhibits the electron transfer in photosystem II (PSII), a key process in the photosynthesis of plants . The herbicide binds to the D1 protein of the PSII reaction center, blocking electron transfer to plastoquinone . This interaction disrupts the conversion of absorbed light energy into electrochemical energy, leading to the production of reactive oxygen species .
Cellular Effects
This compound has significant effects on various types of cells and cellular processes. It induces oxidative stress, damaging the proteins and membranes of photosynthetic cells and leading to cell death . This compound also influences cell function by disrupting photosynthesis, which can impact cell signaling pathways, gene expression, and cellular metabolism .
Molecular Mechanism
The molecular mechanism of action of this compound involves its binding interactions with biomolecules and its influence on enzyme activity and gene expression. As a PSII inhibitor, this compound binds to the D1 protein, blocking electron transfer and disrupting photosynthesis . This leads to the production of reactive oxygen species, which can cause further cellular damage .
Temporal Effects in Laboratory Settings
In laboratory settings, the effects of this compound change over time. Hydrolysis and photolysis of this compound have been examined under various conditions . The herbicide was shown to be stable in incurred samples of liver after 316 days and kidney after 305 days in frozen storage . This indicates that this compound has long-term effects on cellular function.
Dosage Effects in Animal Models
The effects of this compound vary with different dosages in animal models. The 2012 Meeting established an acceptable daily intake (ADI) for this compound of 0–0.09 mg/kg body weight . High doses of this compound could potentially lead to toxic or adverse effects .
Metabolic Pathways
This compound is involved in several metabolic pathways. It is metabolized by the cytochrome P450 gene CYP81A6 . The major metabolites formed are the glycosyl conjugates of 6-hydroxybentazon and 8-hydroxybentazon .
Transport and Distribution
This compound is transported and distributed within cells and tissues. Most herbicides, including this compound, move across plant membranes via nonfacilitated diffusion . The herbicide’s lipophilicity and acidity, along with the plant cell membranes and the electrochemical potential in the plant cell, control its absorption and accumulation .
Subcellular Localization
This compound is localized in the endoplasmic reticulum . This subcellular localization affects its activity and function. The endoplasmic reticulum is involved in the synthesis and metabolism of lipids and proteins, which could be influenced by the presence of this compound .
Preparation Methods
The synthesis of AMG925 involves an eight-step process, which includes several key reactions such as nucleophilic aromatic substitution, iodination, regioselective deprotonation, transmetallation, Negishi coupling, and Buchwald reaction. The final product is obtained through a series of crystallizations and purifications to ensure high purity .
Nucleophilic Aromatic Substitution: This step involves the reaction of a pyrimidine aniline with a fluoropyridine in the presence of potassium bicarbonate and n-butyl alcohol at 110°C.
Iodination: The intermediate product is iodinated using N-iodosuccinimide in 2-methyltetrahydrofuran at 30°C.
Regioselective Deprotonation and Transmetallation: The iodinated product undergoes regioselective deprotonation with n-butylmagnesium lithium in tetrahydrofuran at -10°C, followed by transmetallation with zinc bromide at -2°C.
Negishi Coupling: The resulting zinc reagent is coupled with the iodinated intermediate using palladium tetrakis(triphenylphosphine) in tetrahydrofuran at 65°C.
Cyclization: The biaryl product undergoes an intramolecular nucleophilic aromatic substitution reaction in the presence of potassium tert-butoxide in N-methyl-2-pyrrolidone at 30-75°C.
Buchwald Reaction: The cyclized product is coupled with a chloropyridine using palladium acetate and BrettPhos ligand in isopropyl alcohol at 60°C.
Boc Deprotection: The protected amine is deprotected using hydrochloric acid in isopropyl alcohol at 80°C.
Amide Formation and Salt Formation: The final product is obtained through amide formation using N,N’-diisopropylcarbodiimide and Oxyma in N-methyl-2-pyrrolidone at 60°C, followed by salt formation with acetic acid and hydrochloric acid.
Chemical Reactions Analysis
AMG925 undergoes various types of chemical reactions, including:
Oxidation and Reduction: These reactions involve the gain or loss of electrons, respectively. Common reagents used in these reactions include hydrogen peroxide for oxidation and sodium borohydride for reduction.
Substitution: This reaction involves the replacement of one functional group with another. Common reagents include halogens and nucleophiles.
Cyclization: This reaction involves the formation of a ring structure.
The major products formed from these reactions include various intermediates that are further purified and crystallized to obtain the final product .
Scientific Research Applications
AMG925 has shown significant potential in the treatment of acute myeloid leukemia, particularly in overcoming resistance to Fms-like tyrosine kinase 3 inhibitors. It has demonstrated antitumor activity in various models of acute myeloid leukemia and has been shown to inhibit the growth of Fms-like tyrosine kinase 3-mutated cells .
Its dual inhibition of Fms-like tyrosine kinase 3 and cyclin-dependent kinase 4 makes it a valuable tool for investigating the molecular mechanisms underlying cell proliferation and survival .
Comparison with Similar Compounds
AMG925 is unique in its dual inhibition of Fms-like tyrosine kinase 3 and cyclin-dependent kinase 4, which sets it apart from other inhibitors that target only one of these kinases. Similar compounds include:
Sorafenib: A multikinase inhibitor that targets Fms-like tyrosine kinase 3, vascular endothelial growth factor receptor, and platelet-derived growth factor receptor.
Quizartinib (AC220): A selective inhibitor of Fms-like tyrosine kinase 3.
Palbociclib (PD0332991): A selective inhibitor of cyclin-dependent kinase 4 and cyclin-dependent kinase 6
AMG925 has shown the potential to overcome resistance to Fms-like tyrosine kinase 3 inhibitors, making it a promising candidate for the treatment of acute myeloid leukemia .
Biological Activity
Bentazon methyl, a derivative of the herbicide bentazon, is primarily recognized for its selective herbicidal properties against broadleaf weeds and yellow nut sedge. This article reviews the biological activity of this compound, focusing on its degradation, toxicity, and metabolic effects in various organisms.
Chemical Structure and Properties
This compound is chemically identified as 3-isopropyl-1H-2,1,3-benzothiadiazin-4(3H)-one 2,2-dioxide . It is a systemic herbicide that acts by inhibiting photosynthesis in target plants. The compound's solubility and stability in soil are critical for its effectiveness and environmental impact.
Microbial Degradation
Research indicates that bentazon undergoes microbial degradation in soil, with variations based on tillage practices. A study demonstrated that non-tillage (NT) soils exhibited a more rapid mineralization of bentazon compared to conventional tillage (CT) soils. Specifically, after 12 days, only 15.1% of bentazon remained in NT Dundee soil versus 33.9% in CT soil .
Table 1: Bentazon Degradation Rates in Different Soils
Soil Type | Days After Application | Percentage Remaining |
---|---|---|
NT Dundee | 12 | 15.1% |
CT Dundee | 12 | 33.9% |
NT Miami | 12 | 40.5% |
CT Miami | 12 | 57.4% |
The persistence of bentazon metabolites such as methylbentazon suggests that these compounds may have longer-lasting effects in the environment .
Acute Toxicity
This compound has been evaluated for its acute toxicity across various species. For instance, studies have shown that it exhibits significant toxicity to aquatic organisms, with an EC50 value of 37.7 mg/L for the green algae Pseudokirchneriella subcapitata after 72 hours .
Table 2: Toxicity Data for this compound
Organism | Endpoint | Duration | EC50 (mg/L) |
---|---|---|---|
Pseudokirchneriella subcapitata | Growth Rate (Cell Count) | 72 h | 37.7 |
Pseudokirchneriella subcapitata | Biomass Growth | 72 h | 15.8 |
The data indicate that while this compound is less toxic to mammals (classified as a Group E carcinogen), it poses risks to aquatic ecosystems .
Metabolic Effects on Plants
This compound's impact extends to metabolic processes within plants. A study highlighted its role in enhancing the tolerance of crops like rice to other herbicides such as cloransulam-methyl and halosulfuron-methyl, reducing injury levels significantly when co-applied . This safening effect allows for integrated weed management strategies that can minimize crop damage while effectively controlling weed populations.
Case Study: Rice Tolerance Mechanism
In rice, the expression of cytochrome P450 CYP81A6 has been linked to tolerance against bentazon and metsulfuron-methyl. This gene's expression allows rice plants to metabolize these herbicides more effectively, thereby enhancing their survival rates during herbicide application .
Properties
IUPAC Name |
1-methyl-2,2-dioxo-3-propan-2-yl-2λ6,1,3-benzothiadiazin-4-one | |
---|---|---|
Source | PubChem | |
URL | https://pubchem.ncbi.nlm.nih.gov | |
Description | Data deposited in or computed by PubChem | |
InChI |
InChI=1S/C11H14N2O3S/c1-8(2)13-11(14)9-6-4-5-7-10(9)12(3)17(13,15)16/h4-8H,1-3H3 | |
Source | PubChem | |
URL | https://pubchem.ncbi.nlm.nih.gov | |
Description | Data deposited in or computed by PubChem | |
InChI Key |
XFTQFXBQDVWOCY-UHFFFAOYSA-N | |
Source | PubChem | |
URL | https://pubchem.ncbi.nlm.nih.gov | |
Description | Data deposited in or computed by PubChem | |
Canonical SMILES |
CC(C)N1C(=O)C2=CC=CC=C2N(S1(=O)=O)C | |
Source | PubChem | |
URL | https://pubchem.ncbi.nlm.nih.gov | |
Description | Data deposited in or computed by PubChem | |
Molecular Formula |
C11H14N2O3S | |
Source | PubChem | |
URL | https://pubchem.ncbi.nlm.nih.gov | |
Description | Data deposited in or computed by PubChem | |
DSSTOX Substance ID |
DTXSID80346332 | |
Record name | N-Methylbentazon | |
Source | EPA DSSTox | |
URL | https://comptox.epa.gov/dashboard/DTXSID80346332 | |
Description | DSSTox provides a high quality public chemistry resource for supporting improved predictive toxicology. | |
Molecular Weight |
254.31 g/mol | |
Source | PubChem | |
URL | https://pubchem.ncbi.nlm.nih.gov | |
Description | Data deposited in or computed by PubChem | |
CAS No. |
61592-45-8 | |
Record name | N-Methylbentazon | |
Source | CAS Common Chemistry | |
URL | https://commonchemistry.cas.org/detail?cas_rn=61592-45-8 | |
Description | CAS Common Chemistry is an open community resource for accessing chemical information. Nearly 500,000 chemical substances from CAS REGISTRY cover areas of community interest, including common and frequently regulated chemicals, and those relevant to high school and undergraduate chemistry classes. This chemical information, curated by our expert scientists, is provided in alignment with our mission as a division of the American Chemical Society. | |
Explanation | The data from CAS Common Chemistry is provided under a CC-BY-NC 4.0 license, unless otherwise stated. | |
Record name | Bentazon methyl | |
Source | ChemIDplus | |
URL | https://pubchem.ncbi.nlm.nih.gov/substance/?source=chemidplus&sourceid=0061592458 | |
Description | ChemIDplus is a free, web search system that provides access to the structure and nomenclature authority files used for the identification of chemical substances cited in National Library of Medicine (NLM) databases, including the TOXNET system. | |
Record name | N-Methylbentazon | |
Source | EPA DSSTox | |
URL | https://comptox.epa.gov/dashboard/DTXSID80346332 | |
Description | DSSTox provides a high quality public chemistry resource for supporting improved predictive toxicology. | |
Record name | BENTAZON METHYL | |
Source | FDA Global Substance Registration System (GSRS) | |
URL | https://gsrs.ncats.nih.gov/ginas/app/beta/substances/23AT9KEA2R | |
Description | The FDA Global Substance Registration System (GSRS) enables the efficient and accurate exchange of information on what substances are in regulated products. Instead of relying on names, which vary across regulatory domains, countries, and regions, the GSRS knowledge base makes it possible for substances to be defined by standardized, scientific descriptions. | |
Explanation | Unless otherwise noted, the contents of the FDA website (www.fda.gov), both text and graphics, are not copyrighted. They are in the public domain and may be republished, reprinted and otherwise used freely by anyone without the need to obtain permission from FDA. Credit to the U.S. Food and Drug Administration as the source is appreciated but not required. | |
Retrosynthesis Analysis
AI-Powered Synthesis Planning: Our tool employs the Template_relevance Pistachio, Template_relevance Bkms_metabolic, Template_relevance Pistachio_ringbreaker, Template_relevance Reaxys, Template_relevance Reaxys_biocatalysis model, leveraging a vast database of chemical reactions to predict feasible synthetic routes.
One-Step Synthesis Focus: Specifically designed for one-step synthesis, it provides concise and direct routes for your target compounds, streamlining the synthesis process.
Accurate Predictions: Utilizing the extensive PISTACHIO, BKMS_METABOLIC, PISTACHIO_RINGBREAKER, REAXYS, REAXYS_BIOCATALYSIS database, our tool offers high-accuracy predictions, reflecting the latest in chemical research and data.
Strategy Settings
Precursor scoring | Relevance Heuristic |
---|---|
Min. plausibility | 0.01 |
Model | Template_relevance |
Template Set | Pistachio/Bkms_metabolic/Pistachio_ringbreaker/Reaxys/Reaxys_biocatalysis |
Top-N result to add to graph | 6 |
Feasible Synthetic Routes
Q1: How is N-Methylbentazon metabolized in the environment?
A: Research indicates that N-Methylbentazon, alongside other bentazon derivatives like 8-hydroxybentazon and 8-methoxybentazon, undergoes microbial transformation in the soil. [] This microbial activity, essential for bentazon mineralization, requires oxygen and can be influenced by the presence of additional carbon sources. [] For instance, powdered alfalfa residues were found to impede bentazon mineralization. []
Q2: How does the adsorption of N-Methylbentazon compare to bentazon and its other metabolites?
A: Studies employing various adsorbents, including reversed-phase C18, revealed that N-Methylbentazon exhibits 100% retention on the C18 phase regardless of pH. [] This contrasts with other bentazon metabolites, which showed retention below 40%. [] This suggests that N-Methylbentazon may have a higher affinity for hydrophobic environments compared to other metabolites.
Q3: Are there analytical methods specifically designed to detect N-Methylbentazon?
A: While not specifically mentioned for N-Methylbentazon, an Enzyme-Linked Immunosorbent Assay (ELISA) has been developed for the detection of bentazon and its derivatives. [] This method, validated using gas chromatography, demonstrated high sensitivity, detecting N-methylated bentazon at concentrations as low as 0.01 to 0.1 pM. [] This suggests the potential for adapting similar ELISA techniques for the specific detection and quantification of N-Methylbentazon.
Q4: What is the role of 8-hydroxybentazon in the environmental fate of bentazon?
A: 8-hydroxybentazon appears to be a key metabolite in the formation of bound residues from bentazon in the soil. [] Studies show that the fungus Phanerochaete chrysosporium and the enzyme laccase can convert 8-hydroxybentazon into a dimeric derivative. [] This dimer, along with monomeric 8-hydroxybentazon, contributes significantly to the formation of bound bentazon residues, influencing its long-term persistence in the environment. []
Disclaimer and Information on In-Vitro Research Products
Please be aware that all articles and product information presented on BenchChem are intended solely for informational purposes. The products available for purchase on BenchChem are specifically designed for in-vitro studies, which are conducted outside of living organisms. In-vitro studies, derived from the Latin term "in glass," involve experiments performed in controlled laboratory settings using cells or tissues. It is important to note that these products are not categorized as medicines or drugs, and they have not received approval from the FDA for the prevention, treatment, or cure of any medical condition, ailment, or disease. We must emphasize that any form of bodily introduction of these products into humans or animals is strictly prohibited by law. It is essential to adhere to these guidelines to ensure compliance with legal and ethical standards in research and experimentation.