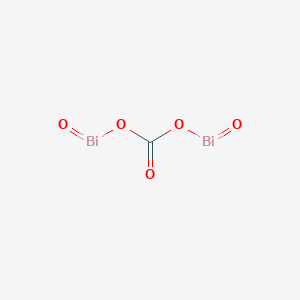
Bismuth subcarbonate
- Click on QUICK INQUIRY to receive a quote from our team of experts.
- With the quality product at a COMPETITIVE price, you can focus more on your research.
Overview
Description
Bismuth subcarbonate, also known as bismuth oxycarbonate or bismuthyl carbonate, is a chemical compound with the formula (BiO)₂CO₃. It contains both oxide and carbonate anions, with bismuth in the +3 oxidation state. This compound occurs naturally as the mineral bismutite and is known for its light-sensitive properties. This compound is a fine white to pale yellow-white powder that is insoluble in water .
Mechanism of Action
Target of Action
Bismuth subcarbonate, a compound of bismuth containing both oxide and carbonate anions , is primarily used to treat ulcers caused by Helicobacter pylori and other gastrointestinal ailments . The primary targets of this compound are the bacterial pathogens, including H. pylori, Escherichia coli, Salmonella, Campylobacter jejuni, and Clostridium difficile .
Mode of Action
It is known that bismuth-based drugs form a protective coating on the ulcer crater, which prevents the erosion generated by gastric acid and contributes to the healing of the ulcer . Bismuth interacts with other anions and compounds, such as hydrochloric acid, bicarbonate, phosphate, and hydrogen sulfide, in the gastrointestinal tract to form bismuth salts such as bismuth oxychloride, this compound, bismuth phosphate, and bismuth sulfide . These bismuth salts possess bactericidal and antimicrobial activity .
Biochemical Pathways
It is known that bismuth-based drugs can inhibit the growth of bacterial pathogens, thereby disrupting their metabolic pathways . More research is needed to fully understand the biochemical pathways affected by this compound.
Pharmacokinetics
It is known that bismuth compounds display multicompartment pharmacokinetics, with the shortest half-life described in humans being 3.5 minutes, and the longest 17 to 22 years . Bismuth compounds may act both locally within the gastrointestinal tract and systemically as a result of gastrointestinal absorption .
Result of Action
The primary result of this compound’s action is the alleviation of gastrointestinal ailments, including ulcers caused by H. pylori . It forms a protective coating on the ulcer crater, preventing further erosion by gastric acid and promoting healing . Moreover, this compound exhibits antibacterial properties, inhibiting the growth of bacterial pathogens .
Action Environment
The action of this compound can be influenced by various environmental factors. For instance, the high pH and high temperature of the aqueous solution can help reduce the time of synthesis of this compound . Moreover, the presence of other anions and compounds in the gastrointestinal tract can influence the formation of bismuth salts and thus the compound’s action .
Biochemical Analysis
Biochemical Properties
Bismuth Subcarbonate interacts with various biomolecules in biochemical reactions. It has been found to have antimicrobial, antiviral, and antiparasitic activity . The compound’s low cost, safety for human use, and effectiveness against various ailments make it a valuable substance in the field of biochemistry .
Cellular Effects
The effects of this compound on cells are diverse and significant. It has been found to be effective against tumoral cells, leishmaniasis, fungi, and viruses . The compound’s bioavailability in physiological environments is limited, which has led to interest in developing new Bismuth compounds and approaches to overcome this challenge .
Molecular Mechanism
At the molecular level, this compound exerts its effects through various mechanisms. It has been found to have potent activity and low toxicity, making it a desirable candidate for developing new drugs .
Temporal Effects in Laboratory Settings
In laboratory settings, the effects of this compound change over time. The compound’s stability, degradation, and long-term effects on cellular function are areas of ongoing study .
Metabolic Pathways
This compound is involved in various metabolic pathways. It interacts with several enzymes and cofactors, and can affect metabolic flux or metabolite levels .
Transport and Distribution
The transport and distribution of this compound within cells and tissues are complex processes. The compound can interact with various transporters or binding proteins, and can affect its localization or accumulation .
Subcellular Localization
The compound may be directed to specific compartments or organelles by targeting signals or post-translational modifications .
Preparation Methods
Synthetic Routes and Reaction Conditions: Bismuth subcarbonate can be synthesized through various methods. One common method involves the reaction between bismuth nanoparticles and atmospheric carbon dioxide dissolved in water. This reaction tends to form nanoplates or small round nanospheres when grown in the presence of halloysite nanotubes . Another method involves the reaction of bismuth nitrate with sodium carbonate under controlled pH and temperature conditions. The reaction is typically carried out at a pH of 8.5-9.0 and a temperature of 50-55°C for about 40 minutes .
Industrial Production Methods: Industrial production of this compound often involves the use of industrial-grade sodium carbonate and bismuth nitrate. The process includes the removal of heavy metals through absorption using modified polyacrylamide-co-styrene-co-acrylic acid copolymer. The reaction conditions are carefully controlled to ensure high purity and yield of this compound .
Chemical Reactions Analysis
Types of Reactions: Bismuth subcarbonate undergoes various chemical reactions, including:
Oxidation: this compound can be oxidized to form bismuth oxide.
Reduction: It can be reduced to elemental bismuth under certain conditions.
Substitution: this compound can react with acids to form bismuth salts and carbon dioxide.
Common Reagents and Conditions:
Oxidation: Typically involves the use of oxidizing agents such as oxygen or hydrogen peroxide.
Reduction: Reducing agents like hydrogen or carbon monoxide can be used.
Substitution: Acids such as hydrochloric acid or sulfuric acid are commonly used in substitution reactions.
Major Products:
Oxidation: Bismuth oxide.
Reduction: Elemental bismuth.
Substitution: Bismuth salts and carbon dioxide.
Scientific Research Applications
Bismuth subcarbonate has a wide range of applications in scientific research:
Comparison with Similar Compounds
Bismuth subsalicylate: Used as an antidiarrheal and anti-inflammatory agent.
Bismuth oxychloride: Used in cosmetics and pigments.
Bismuth citrate: Used in medical applications for its antibacterial properties.
Uniqueness: Bismuth subcarbonate is unique due to its combination of oxide and carbonate anions, which imparts distinct chemical and physical properties. Its light-sensitive nature and ability to form nanostructures make it particularly valuable in scientific research and industrial applications .
Biological Activity
Bismuth subcarbonate, chemically represented as (BiO)2CO3, is a compound that has garnered attention for its diverse biological activities, particularly in the fields of antimicrobial and anticancer research. This article provides a comprehensive overview of the biological activity of this compound, supported by recent research findings, case studies, and data tables.
Overview of this compound
This compound is primarily utilized in medical applications due to its ability to treat gastrointestinal disorders, particularly those associated with Helicobacter pylori infections. Its mechanism of action involves multiple pathways, including enzyme inhibition and disruption of bacterial adhesion.
Recent studies have elucidated several mechanisms through which this compound exerts its biological effects:
-
Antimicrobial Activity :
- Bismuth compounds, including this compound, have demonstrated significant antibacterial activity against H. pylori. This activity is attributed to the inhibition of key enzymes such as urease and F1-ATPase, which are crucial for bacterial survival and metabolism .
- The compound disrupts the oxidative defense systems in H. pylori, leading to increased susceptibility to oxidative stress .
-
Anticancer Properties :
- Bismuth has been investigated for its potential anticancer properties, with studies indicating that it can interact with nucleotides and amino acids in enzymes, potentially leading to apoptosis in cancer cells .
- The compound's ability to bind to proteins involved in cancer progression has been highlighted, suggesting a multifaceted approach to cancer treatment .
- Electrochemical Applications :
Case Study 1: Antibacterial Efficacy Against H. pylori
A study by Sun et al. demonstrated that bismuth compounds inhibit H. pylori growth by downregulating virulence factors such as CagA and VacA. The researchers employed proteomic and metabolomic analyses to reveal that bismuth treatment disrupted metabolic pathways critical for bacterial growth .
Case Study 2: Anticancer Activity
In a study focusing on the anticancer potential of bismuth compounds, researchers found that this compound exhibited cytotoxic effects on various cancer cell lines. The mechanism was linked to the induction of oxidative stress and disruption of mitochondrial function, leading to apoptosis .
Data Tables
The following table summarizes key findings related to the biological activity of this compound:
Study | Activity | Mechanism | Findings |
---|---|---|---|
Sun et al., 2023 | Antibacterial | Inhibition of urease and F1-ATPase | Downregulation of CagA and VacA |
Yao et al., 2023 | Antibacterial | Disruption of oxidative defense | Inhibition of flagella assembly |
Research on Cancer Cells | Anticancer | Induction of oxidative stress | Apoptosis in various cancer cell lines |
Kefala et al., 2020 | Electrochemical Sensor | Enhanced sensitivity through nanocomposite formation | Successful detection of cadmium and lead |
Properties
CAS No. |
5892-10-4 |
---|---|
Molecular Formula |
CBiO4-2 |
Molecular Weight |
284.99 g/mol |
IUPAC Name |
bis(oxobismuthanyl) carbonate |
InChI |
InChI=1S/CH2O3.Bi.O/c2-1(3)4;;/h(H2,2,3,4);;/p-2 |
InChI Key |
GUZOHYPCAAYGEN-UHFFFAOYSA-L |
SMILES |
C(=O)(O[Bi]=O)O[Bi]=O |
Canonical SMILES |
C(=O)([O-])[O-].[O].[Bi] |
Key on ui other cas no. |
5892-10-4 |
physical_description |
Dry Powder White powder; [Alfa Aesar MSDS] |
Synonyms |
Bismuth Carbonate Oxide; (μ-Carbonato)dioxodibismuth; 1,3,5-Trioxo-2,4-Dioxa-1,5-dibismapentane; Bismuth Oxide Carbonate ((BiO)2CO3); Bismuth Oxycarbonate; Bismuth Oxycarbonate (Bi2O2CO3); Bismuth Subcarbonate; [Carbonylbis(oxy)]bis[oxobismuthine; Bi |
Origin of Product |
United States |
Retrosynthesis Analysis
AI-Powered Synthesis Planning: Our tool employs the Template_relevance Pistachio, Template_relevance Bkms_metabolic, Template_relevance Pistachio_ringbreaker, Template_relevance Reaxys, Template_relevance Reaxys_biocatalysis model, leveraging a vast database of chemical reactions to predict feasible synthetic routes.
One-Step Synthesis Focus: Specifically designed for one-step synthesis, it provides concise and direct routes for your target compounds, streamlining the synthesis process.
Accurate Predictions: Utilizing the extensive PISTACHIO, BKMS_METABOLIC, PISTACHIO_RINGBREAKER, REAXYS, REAXYS_BIOCATALYSIS database, our tool offers high-accuracy predictions, reflecting the latest in chemical research and data.
Strategy Settings
Precursor scoring | Relevance Heuristic |
---|---|
Min. plausibility | 0.01 |
Model | Template_relevance |
Template Set | Pistachio/Bkms_metabolic/Pistachio_ringbreaker/Reaxys/Reaxys_biocatalysis |
Top-N result to add to graph | 6 |
Feasible Synthetic Routes
Disclaimer and Information on In-Vitro Research Products
Please be aware that all articles and product information presented on BenchChem are intended solely for informational purposes. The products available for purchase on BenchChem are specifically designed for in-vitro studies, which are conducted outside of living organisms. In-vitro studies, derived from the Latin term "in glass," involve experiments performed in controlled laboratory settings using cells or tissues. It is important to note that these products are not categorized as medicines or drugs, and they have not received approval from the FDA for the prevention, treatment, or cure of any medical condition, ailment, or disease. We must emphasize that any form of bodily introduction of these products into humans or animals is strictly prohibited by law. It is essential to adhere to these guidelines to ensure compliance with legal and ethical standards in research and experimentation.